Water, arguably one of the most important “nutrients” in the diet, despite having little in the way of nutrient content. We have all heard that water (fluid) makes up a significant portion of our body and is vital to human survival. However, there is still a lot of conjecture in the literature, and of course online, as to how much we actually need.
Hydration is defined by the Oxford Dictionary (n.d.) as the “process of causing something to absorb water”. Even this dictionary knows the importance of hydration to the human body as its example of using hydration in a sentence is “the human body requires adequate hydration to function properly". Bravo Oxford Dictionary!
In this topic, we will cover:
- Various types of fluids for hydration
- The role of water in hydration
- When and where to use or avoid each type of fluid
- The consequences of ineffective hydration on health and performance.
Additionally, we will discuss hydration strategies for different sporting events and environments using what the research tells us about fluid losses in these situations.
Key terms
Before getting started, let’s familiarise ourselves with some common terms.
Click on each word to reveal its description.
Essentially, these are bodily fluids that are not contained inside cells. This includes the fluid found in blood (plasma), the lymphatic system, in body cavities that are lined with serous (moisture-exuding) membranes (e.g., saliva glands), around the intestines, brain and spinal cord (Britannica, 2021).
Extracellular fluid comprises approximately 20% of total body weight (this is around a third of total fluid weight). Of this, around 5% of extra-cellular fluid is blood plasma, with 12% found around the intestines, brain, spinal cord and within the lymphatic system. The rest is produced by secreting glands and membranes (Brinkman, Dorius and Sharma, 2023).
Acids, bases and salts that include but are not limited to sodium, potassium and calcium. These activate enzymes to control various metabolic processes or generate nerve impulses. Different fluids within the body contain different amounts of these substances dependent on their function. For example, extracellular fluid tends to be higher in sodium, chloride, bicarbonate, and proteins (Brinkman, Dorius and Sharma, 2023). While water contains limited amounts of electrolytes (compared to the body fluids it maintains), other fluids we drink often contain electrolytes (e.g. sports drinks, milk etc).
An agent which increases urine production and excretion. These substances can be found in popular drinks and can lead to increased urine production depending on an individual’s sensitivity to them. While tea and coffee are considered mild diuretics which appear to mimic the effects of dehydration (without substantially dehydrating the body), energy drinks with large amounts of caffeine and alcoholic beverages are considered potent diuretics. It has also been suggested that drinks containing high amounts of sugar, can limit the water that your digestive system absorbs having a dehydrating effect (Aven, 2007 (Conley, 2018).
Dehydration occurs when you lose more fluid from your body than you take in. It is essentially a physical state where your body doesn’t have enough fluid to carry out its functions optimally. Of course, the body will continue to perform most functions in a dehydrated state, however, this often involves placing more stress on organs and a redirection of fluid from one part of the body to another to take care of the greatest need. This will often result in negative effects in the areas the fluid has been borrowed from.
Prepare for assessment
Prepare yourself for the upcoming assessment by familiarising yourself with Assessment 2A.
- Review the requirements provided to gain a clear understanding of what is expected.
- Manage your time carefully to work on this topic and assessment for a total of a 1-week period.
- Aim to complete and submit your assessment at the completion of this topic.
While the difference between these terms seems obvious, the distinction between water and fluid is important. These terms are often used interchangeably, but they are most definitely not the same thing.
Fluid is a broad term encompassing any substance that flows easily because of increased molecular space and because they don’t have a fixed shape. Both liquids and gases are considered fluids (Britannica, 2021). In terms of relevance to the human body, fluid would encompass anything we drink in liquid form. Therefore, water is a fluid. During this topic, fluid will also be used to refer to a number of substances found within the human body (e.g. blood, saliva, etc).
Water is a colourless, transparent, odourless liquid that forms the seas, lakes, rivers, and rain and is the basis of the fluids of living organisms (Oxford Dictionary, n.d.). In other words, water makes up most fluids. One of the key distinctions between water and fluids in the topic of hydration is that water is always hydrating, whereas the same cannot be said for all fluids.
Before diving into this topic, there are a few other terms that will be useful for you to understand as they will be referred to often throughout the topic.
Maintaining optimal fluid levels in the body is critical to the normal functioning of most bodily processes. Proper hydration is essential for:
- Regulating body temperature
Blood plasma plays a critical role in the removal of excess heat from the internal system to the skin where it is released. - Optimal joint function
It helps joints by lubricating them in the form of synovial fluid. Cartilage is also around 80% water, so being hydrated ensures optimal cushioning between bones. - Transportation of nutrients and oxygen through the body
Through maintaining the proper consistency of blood. Reduced blood volume through dehydration also places unnecessary stress on your heart, which has to work harder to circulate blood. - Removal of waste products and toxins
By allowing the regular release of fluid in urine and faeces - Proper functioning of sensory organs relating to sight, smell, and taste
Secretions of fluid in the nose, mouth, and eyes lead to enhanced function of these senses. - Proper functioning of the brain
Even mild dehydration affects memory, mood, concentration, and reaction time. This is likely due to both a reduction in cerebral and spinal fluid and a reduction in oxygen delivery to the brain due to reduced blood volume. - Proper digestive function
Your body needs water in order to digest food properly. Dehydration can lead to irregular bowel movements, gas, bloating, and heartburn. - Biochemical reactions and nutrient formation
All biochemical reactions occur in water. It fills the spaces in and between cells and helps form structures of large molecules such as protein and glycogen.
Achieving water balance
How much water do I need to drink every day for optimal hydration?
This is a huge area of debate because there is yet to be any consistent evidence proving that a particular amount of water improves health or reduces the risk of disease. A quick internet search yields a variety of answers and even a more detailed search of scientific research does not give any clarity.
Established facts
In someone who leads a fairly sedentary lifestyle (i.e., is not involved in vigorous daily activity) total fluid losses per day for a 70kg adult averages between 2.5L-3L (Nutrient Reference Values for Australia and NZ, 2006). Fluid exits the body in a number of ways. The main ways include:
- Urine.
- Faeces.
- Sweat.
- Breathing.
50% of water turnover in our body is “insensible losses” through breathing and the skin. These are sensitive to environmental conditions and exercise, i.e. they are increased during hotter temperatures and during activity. The remainder is lost in urine and faeces (Nutrient Reference Values for Australia and NZ, 2006).
These fluid losses must be balanced with fluid input to remain hydrated. Our tissues produce a modest amount of fluid (around 300ml) per day as a waste product of basic metabolism under normal non-exercise conditions. The remaining fluid deficit must therefore be balanced by drinking fluids or eating fluid-containing foods. The average water consumption yielded from solid food per day is around 700ml (Human Nutrition, 2017).
Obviously, this figure is highly variable and dependent on the types of food you eat as some foods contain more water weight than others. Here are some examples of common foods and the percentage of water content. As you can see fruits and vegetables have the highest water content, while nuts crackers, and oils contain the least. Note: oil is a fluid, but not water.
The Water Content Range for Selected Foods | |
---|---|
Percentage | Food Item |
100% | Water |
90–99% | Fat-free milk, cantaloupe, strawberries, watermelon, lettuce, cabbage, celery, spinach, pickles, squash (cooked) |
80–89% | Fruit juice, yogurt, apples, grapes, oranges, carrots, broccoli (cooked), pears, pineapple |
70–79% | Bananas, avocados, cottage cheese, ricotta cheese, potato (baked), corn (cooked), shrimp |
60–69% | Pasta, legumes, salmon, ice cream, chicken breast |
50–59% | Ground beef, hot dogs, feta cheese, tenderloin steak (cooked) |
40–49% | Pizza |
30–39% | Cheddar cheese, bagels, bread |
20–29% | Pepperoni sausage, cake, biscuits |
10–19% | Butter, margarine, raisins |
1–9% | Walnuts, peanuts (dry roasted), chocolate chip cookies, crackers, cereals, pretzels, taco shells, peanut butter |
0% | Oils, sugars |
Source: The USDA National Nutrient Database for Standard Reference, Release 21 provided in Altman.
Table source: https://www.ncbi.nlm.nih.gov/pmc/articles/PMC2908954/table/T1/?report=objectonly
Let's consider the numbers…
If your daily water requirement is 2.5L...
your body naturally produces 300ml...
and you gain 700ml from food...
This leaves around 1.5L to be consumed as fluids to stay hydrated.
But is that the definitive answer? Let's delve deeper.
Water requirements vary significantly due to various factors such as metabolism, diet, climate, and clothing. As a result, maintaining proper hydration is achievable with a diverse range of fluid intakes. It's important to note that human water needs should not be solely determined by a "minimal" intake, like the 2.5L figure, as this approach could potentially result in a deficit and lead to unfavourable performance and health outcomes. (Sawka et al, 2005).
Numerous suggestions have been put forward by a number of National government health bodies and Nutritional Authorities, but almost none of them can agree on an approximate amount. Here are a few of the recommendations from reputable organisations.
Note: these figures are for total water intake including that from food.
Organisation | Recommendations |
---|---|
National Research Council of America | Approximately 1ml/kcal expended in a day. Example: 2000 calorie diet requires 2L fluid. Fluid intake needs to increase with your size and activity level. Note: Scientific data supporting this calculation is limited. |
Food and Nutrition Board of the Institute of Medicine | Infants and babies: 600ml Children: 1.7L Adult Men: 2.5L (sedentary) or 3.2L (moderately active) Adult Women: Limited information is available in the research for women, but the available data suggests that women may require 0.5-1L less fluid daily compared to males. Very active adults and those living in warm climates: Up to 6L a day. |
Harvard University School of Health | 4-6 cups a day is sufficient for most sedentary adults, adjust for activity and temperature. |
National Academy of Medicine | Women: 2.7L of fluid a day (from food and drink). Men: 3.7L of fluid a day (from food and drink). |
European Hydration Institute | Females: 2.0L/day Males: 2.5L/day, This was discussed by a panel of experts in 2010. Note: The panel also recommended that these figures include the elderly despite lower energy requirement. This is because the elderly have a decrease in renal concentrating capacity. |
Fluid recommendations for New Zealand
The recommendations for fluid intake for NZ and Australian populations were derived from the National Nutrition Survey of Australia (1995). The Average Intake (AI) fluid figures for adults are based on the highest level of fluid intake by each age group (which included both alcoholic and non-alcoholic consumption). These recommendations are shown in the table below and state fluid intake requirements that are somewhat higher than those of other countries.
AVERAGE INTAKE FOR ADULTS IN VARIOUS AGE GROUPS | ||
---|---|---|
Total water (Food and fluids) | Fluids (Including plain water, milk and other drinks) | |
Men | ||
19-30 yr | 3.4 L/day | 2.6 L/day (about 10 cups) |
31-50 yr | 3.4 L/day | 2.6 L/day (about 10 cups) |
51-70 yr | 3.4 L/day | 2.6 L/day (about 10 cups) |
>70 yr | 3.4 L/day | 2.6 L/day (about 10 cups) |
Women | ||
19-30 yr | 2.8 L/day | 2.1 L/day (about 8 cups) |
31-50 yr | 2.8 L/day | 2.1 L/day (about 8 cups) |
51-70 yr | 2.8 L/day | 2.1 L/day (about 8 cups) |
>70 yr | 2.8 L/day | 2.1 L/day (about 8 cups) |
Table source: https://www.eatforhealth.gov.au/nutrient-reference-values/nutrients/water
With numerous recommendations available, it's understandable to feel uncertain about which to trust. The most balanced approach likely involves integrating these various suggestions. The 2.5L value seems to fall within a reasonable range of these recommendations, making it a practical starting point. Considering that approximately 20% of fluid intake is derived from food in standard diets, and around 10% through metabolism, accounting for roughly a litre of fluid, aiming for the 6 cups figure (1.5L) appears to be a sensible baseline. This provides a starting point to ensure proper hydration, and adjustments can be made from there as needed.
Evaluating your client's hydration status
During the last 20 years, many methods have been developed to assess hydration levels accurately in humans including:
- Changes in body weight
- Blood and urine analysis
- Bioelectric impedance scans
- Skinfold thickness
- Heart rate tracking
- Blood pressure.
In a clinical setting, the most widely used methods to assess hydration status are:
- Plasma osmolarity (consistency)
- Urine osmolarity.
Urine colour has been employed with reasonable accuracy. This method proves useful when laboratory analysis is unavailable or when a rapid estimate of hydration status is required. Some data indicates that urine colour is as good an indicator of hydration as plasma or urine osmolarity analysis (Kavouras, 2002). Along with changes in body weight, urine colour is a reasonable indicator of hydration status especially when analysis is based on the first morning urine sample (Kavouras, 2002).
Other sources are less enthusiastic about the use of urine colour as a means of assessing hydration. This is because there are many factors that can influence urine colour including diet, drugs, supplements and medication, caffeine, illnesses, the duration over which urine has accumulated in the bladder and age (Barley et al, 2020). For these reasons much of the literature recommends using multiple measures of hydration as no single approach is without limitations.
However, a quantitative analysis performed by Perrier et al (2016) compared urine colour chart assessment against more scientific methods of hydration analysis and concluded that there was a definite quantifiable relationship between a purposeful change in total fluid intake and urine colour, and that colour changes were significantly aligned with more scientific measures.
This gives some confidence that in the absence of more sophisticated measures, the monitoring of body weight and urine colour offers a practical means for the general population to evaluate and adjust hydration behaviours (Perrier et al, 2016).
Checking a urine sample
Pointers for collecting urine samples for assessing hydration:
Pointers for collecting urine samples for assessing hydration:
- Collect the urine in a clear plastic or glass container (nothing that has any sort of colour in it).
- Do not assess urine from the toilet bowl, as that urine has mixed with a considerable amount of clear water
- Collect urine from mid-stream (i.e. halfway through a pee)
- Ensure well-lit conditions
- Be aware that particular vitamin supplements (especially vitamin B) can create a yellowing effect on urine.
- Daily morning weighing (post pee) will also provide an indication of hydration status.
Unfortunately, there are no available statistics related to the NZ public on this issue, however, we are able to draw some conclusions based on evidence from other countries. Most of the literature out of America suggests the average adult is not consuming anywhere near the recommended amounts with the results of multiple surveys reporting that the average American adult only consumes around 2.5 cups of fluid a day (How many people are dehydrated, 2022).
An article by Taylor and Jones (2022) published by the National Library of Medicine suggests that despite a sparsity of medical literature on the subject, it is estimated that up to 75% of Americans are chronically dehydrated.
How many people are dehydrated (2022) produced the following infographic depicting the levels of chronic dehydration in a number of countries. While the evidence to support this is limited, similar figures were reported by other publications and if there is an element of truth to this, then it is likely that a good number of New Zealanders are also chronically dehydrated.
Chronic Dehydration
The human body is an incredible system. Even in situations where it's not provided with what it requires, it manages to continue functioning, albeit potentially at a diminished capacity. Research indicates that a considerable portion of the population might be experiencing chronic dehydration. This suggests that they have consistently not provided their bodies with sufficient fluids for an extended duration, causing their bodies to adjust to this altered state.
The body tells us when we are dehydrated. Yes, the body does produce a multitude of symptoms that alerts us to its needs, but when we don’t listen to these prompts over time, the messages lose their effectiveness. For example, if you notice that you are feeling hungry, but the opportunity to eat something isn’t available, your body will message your brain, but once it is established that no food is available, those messages become dampened. The same is true for fluid intake. Your body will alert you to the fact that you need to drink something. This is often signaled by sensations of thirst or the onset of a mild headache.
However, if you are immersed in something, or don’t have fluid available, these signals can quickly diminish. Bear in mind also, that when you are thirsty, you are already dehydrated (often up to 2% of fluid weight). Having a few sips of water will alleviate the feeling of thirst, but won’t come close to bringing you back to a fully hydrated state. Over time, this becomes a chronic deficiency. It appears that this state can be maintained without too many major issues, but over time a raft of issues will present.
Watch the following short video, the effect of chronic dehydration on the body is simplified using a car analogy.
Now you have an ideal of the ideal amount to drink to stay hydrated. Let's learn about how we should consume it.
Drinking to thirst vs drinking to a plan
There is a growing school of thought that a person simply needs to drink to thirst, and they will avoid dehydration. After all, that is what the thirst mechanism is for. In most instances, thirst and hunger drives combined with easy access to water and food usually off-sets water losses (Kenefick, 2018). However, studies have shown that not everyone’s thirst mechanism operates at the same level. True thirst mechanisms are thought to kick in when a body is around 2% depleted of water mass.
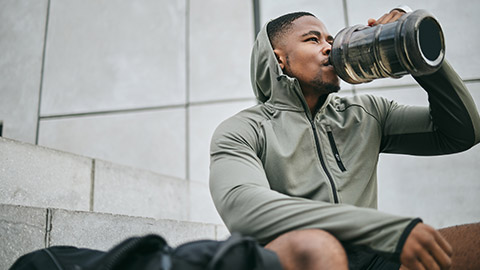
The thirst mechanism is thought to be stimulated by an elevation in plasma osmolarity (thickness) and to a lesser degree reduced plasma volume (Cheuvront et al, 2013). Osmoreceptors detect higher sodium concentration in the blood (due to a concentration/thickening of plasma) and the hypothalamus activates the thirst mechanism and releases anti-diuretic hormone (ADH) which reduces water loss through urine production (Kenefick, 2018).
Conservative estimates suggest the body is at least 60% water, so a 2% loss in body mass equates to over a kilogram of body weight loss for a 70kg person. This is the reason why the drink-to-thirst approach may not be the best to use for the general population. While thirst is a mechanism that tells us we need to drink, thirst tends to be alleviated before complete rehydration is achieved (Kenefick, 2018). For example, you feel thirsty, so you drink something. You alleviate the thirst signal, but likely won’t fully rehydrate (most people don’t drink a litre in one go). Therefore, while the thirst mechanism is switched off, you are still not fully hydrated. This cycle, repeated over time can lead to chronic dehydration. There is also clear evidence that the thirst mechanism gets less effective as we age making older populations even more susceptible to dehydration.
A better approach for the general public is to drink regularly and monitor amounts across the day to ensure you imbibe enough fluid. If a person has not been drinking nearly enough fluids for some time, it is suggested to slowly and consciously increase the amount drunk over days until the desired volume is achieved consistently.
Important
The origin of "drink to thirst" is somewhat unfortunate, as it has been adopted from specific exercising groups and spread to the general public. The concept gained popularity in endurance training circles, particularly to address the concern of excessive water intake during longer events. Traditionally, exercise fluid plans recommended consuming around 150-300ml of fluid every 15 minutes. However, this approach proved excessive for races lasting several hours. Consequently, researchers began investigating the ideal fluid replacement strategy for endurance athletes. As is common in such cases, these studies yielded a range of findings.
Sipping versus sculling
If you've forgotten to drink water for a while, should you quickly consume a couple of glasses to compensate? The answer is no. Drinking a significant amount of water all at once may result in a rapid increase in blood volume as the water rapidly exits the digestive system. This sudden change can make it challenging for the body's tissues to absorb the entirety of the water you've consumed. The kidneys detect the increased volume and interpret it as an excess, leading them to initiate urine production to remove the perceived surplus. To understand this process better, you can watch the following video.
In summary, it's evident that we should aim for a daily fluid intake target, with a minimum of 1.5 litres being recommended. Spacing out this amount consistently throughout the day is advisable. But here's the question: Does this fluid intake specifically need to be water?
Why staying hydrated can be challenging
We now have all the basics sorted for staying hydrated. The approximate amounts we should be drinking, how to drink it, and what to drink. Despite this information not being groundbreaking, many individuals still struggle with staying adequately hydrated and often opt for the wrong fluids. Let’s learn more on what contributes to this challenge.
There are three main reasons why people don’t drink enough water. These are:
1: Water is boring
According to surveys conducted in Britain and the USA, over 50% of respondents don’t drink much water because they find water boring. In a Britvic survey conducted in the UK in 2019, 20% of the participants admit they last drank a glass of water more than a week ago (Billi, 2009). Respondents said they were most often drinking carbonated soft drinks instead of water as they were more “thirst quenching” than water. There is some evidence that cool carbonated drinks appear to quench the thirst response quicker than water, however, this can also lead to a lack of total fluid consumption.
Tips: Drink sparkling water to get the carbonation quench effect.
2: Don’t like the taste
Over 50% of respondents reported that the water from their household taps didn’t taste good, with the majority suggesting chlorination (or chemical tastes) were the issue.
Tips: Improvements in taste can be made without adding significant sugar to water. Commonly used items include:
- Sliced citrus fruits or zest (lemon, lime, orange, grapefruit)
- Crushed fresh mint
- Peeled, sliced fresh ginger or sliced cucumber
- Crushed berries
- Mix a little fruit juice into water (more water than juice).
3: Too busy- forget to drink
A poll of over 2000 UK population members in 2018 found that 75% were so busy they regularly skipped meals and forgot to drink water (Knight, 2018). Only 1 in four were found to be drinking water regularly through the day with most only getting their fluid from tea or coffee. 30% of working aged adults surveyed said they need “caffeine more than water” to function in their workplace (Billi, 2019).
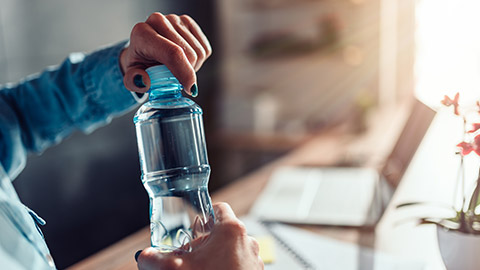
Tips: To improve water intake in the workplace try to make water readily available and visible. This can be by having your own water bottle on the desk, or a water cooler in the office. The next step is setting reminders for yourself to drink. This can be easily done by setting watch or phone reminders, or by using one of many free “drink water” reminder apps.
Try it out
Now, let's put your knowledge to the test. Take a moment to reflect on what we've explored thus far and answer the following questions. If you find any questions challenging, consider revisiting the content to reinforce your understanding.
Dehydration can have a range of negative effects on the body. Even mild dehydration can lead to decreased cognitive function, reduced physical performance, and impaired mood. As dehydration worsens, it can result in symptoms like headaches, dizziness, dry skin, and dark yellow urine. Severe dehydration can lead to more serious complications such as heat-related illnesses, kidney problems, and even fainting. Staying properly hydrated is crucial for maintaining overall well-being and supporting optimal bodily functions. We must educate our clients on why it is important to drink the required daily amount of water.
Read on to learn about the effects of different levels of dehydration on:
- the body’s organs and functions
- exercise performance.
The body’s organs and functions
Understanding the intricate relationship between hydration and organ function is essential for maintaining overall health and well-being. Hydration plays a vital role in supporting the proper functioning of various organs and bodily processes. Let’s take a look at how dehydration affects the:
- heart
- kidneys
- brain
- muscles.
The heart
Dehydration increases the viscosity (thickness) and reduces the volume of blood in your body; thus, the heart has to compensate for this by working harder to distribute the remaining blood to tissues. As stroke volume and cardiac output reduces with dehydration, the body begins to compensate by moving fluid from other spaces into the bloodstream. The depletion of fluid from these regions (such as around the brain and digestive system) clarifies the typical symptoms linked with dehydration, including sensations of dizziness, reduced appetite, and nausea. (Ashraf and Rea, 2017). If you are chronically dehydrated, your heart rate will remain elevated over time, placing unnecessary stress on the heart. More recently, research has indicated that dehydration can affect the ability of blood vessels to vasodilate (widen) which may impact the ability to regulate blood pressure (Watson and Farquhar, 2019).
The kidneys
Dehydration has multiple effects on the kidneys. The kidneys receive less blood flow when we are dehydrated which in turn leads to more concentrated urine with higher concentration of minerals and waste products. This can lead to the formation of crystals in kidneys that can affect kidney function and contribute to certain kidney diseases and kidney stones (Kidney Research UK, n.d.).
Even mild chronic dehydration could be a risk factor associated with all types of chronic kidney dysfunction and disease (Roncal-Jimenez, 2015). There are a number of potential contributing factors, one of which appears to be that dehydration increases the production of vasopressin (a compound that causes kidneys to retain water). Elevated levels of vasopressin have been indicated in causing or exacerbating chronic kidney disease by reducing the efficiency of sodium and urea excretion placing an increased demand on kidneys and by accelerating cyst growth during kidney disease (Bankir et al, 2013).
The brain
Water accounts for 75% of brain mass. A meta-analysis performed by Wittbrodt and Millard-Stafford (2018) examined 33 studies on the area of dehydration and cognitive function and concluded that dehydration impairs cognitive performance, particularly for tasks involving attention, executive decision making and motor co-ordination. These cognitive deficits were noticeably worse when water losses equating to 2% of body mass loss and continued to worsen as the dehydration state worsened. Mills (2020) also reported that women of all ages (but especially elderly women) are more sensitive to the effects of dehydration on cognitive decline. What is also interesting is that in younger people the cognitive effects of dehydration are easily reversed by hydrating, but that in elderly, the effects appear to be cumulative and promote continued cognitive decline (Mills, 2020).
The muscles
Negative effects of dehydration on muscle stem from a reduction in blood volume. This affects muscle in a number of ways. Blood flow to exercising muscles is significantly reduced with dehydration mainly due to reductions in blood pressure and therefore gas exchange pressure at tissues. Dehydration caused by sweating, results in water within muscle cells being transported along with heat to the skins surface leading to muscle cell dehydration and impaired function (Sawka, 1992). Muscle function is likely impaired due to dehydration impeding thermal regulation (less plasma means less ability to remove heat), by the altering of water movement across cell membranes, and by interfering with actin and myosin cross-bridge formation (Cleary et al, 2006).
Exercise performance
Having examined the consequences of dehydration on the body's major organ systems, it becomes evident how dehydration's influence on the cardiovascular, renal, cognitive, and muscular systems could significantly affect exercise performance. However, the extent of dehydration's impact and the equivalence of its effects across various forms of exercise remain to be explored.
Let’s look at how dehydration impacts on:
- endurance performance
- intermittent activity (i.e. sports)
- power athletes and resistance training populations.
Endurance performance
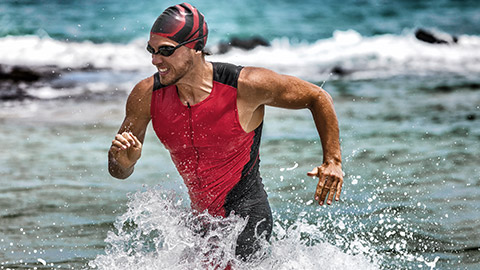
The vast majority of literature on this subject indicates that endurance performance is negatively impacted by dehydration. Additionally, dehydration is common in endurance athletes during training and competition as typically, fluid ingested during prolonged exercise is insufficient to keep pace with sweat losses (James et al, 2019). This appears to be the case even when athletes are fully hydrated when they begin endurance exercises. It is widely accepted that dehydration of around 2% of body mass impairs endurance performance. However, it would appear that losing 2% of body weight is quite easy to do during endurance exercise.
How much sweat do endurance athletes lose?
Barnes et al (2019) conducted a multiple sports analysis of sweat rates and composition to establish some normative data on sweat figures for different sports. Their study included 255 endurance athletes encompassing distance runners, cyclists, and triathletes. The findings of this study showed that in normal summer conditions (with ambient temperatures of between 22 and 30 degrees Celsius with moderate humidity levels) and when working at moderate intensities, these mid-level athletes returned an average sweat loss of 1.28L per hour of exercise. The experiment did however show that there was high variability in sweat rates between athletes with a range from 0.71L - 1.85L of sweat lost per hour shown.
Endurance athletes also appeared to lose more sodium in their sweat from continuous exercise, than those participating in intermittent sports (although this was attributed to the duration of exercise completed). 1.28L of body fluid loss is nearly 2% of a 70kg athlete’s body mass (1.85L constitutes a 2.6% loss of body mass).
Those athletes competing in shorter duration events like a 10km race, will work at higher intensities and therefore produce even higher sweat rates than these average figures. Those athletes involved in longer races (like marathons) often take between 2.5 and 3 hours to complete the race meaning total fluid losses of over meaning total fluid losses of up to 5-6L in a race is not uncommon (7-8% of body mass). Given that elite endurance athletes maintain higher intensities for races, it has been estimated that top runners may lose more like 2.3L of sweat per hour (Hutchinson, 2012).
These sweat rates are of course also affected by ambient temperature and humidity. In 2009 Haile Gebrselassie was weighed before and after winning the 2009 Dubai Marathon. It was found that he lost 9.8% of his starting body weight dropping from 58.2kg at the start of the race, to 52.5kg at the end despite drinking 1.7L of fluid during the race (Hutchinson, 2012).
During a marathon there are typically drink stations every 5km (so 8-9 in total). This means an athlete in the median sweat loss range indicated by the Barnes et al (2019) study would have to drink 500ml of fluid to remain hydrated (and an elite athlete more like 600-700ml). This is obviously too difficult to achieve while running, so becoming dehydrated seems inevitable.
Physiological and perceptual effects
Dehydration appears to impair endurance performance through a combination of mechanisms, however, most of these impairments stem from sweat losses that result in reduced blood plasma volume and consequent increases in osmolarity (concentration) of extracellular fluid. According to James et al (2019), the key physiological issues that directly impact endurance performance include:
- Reductions in muscle and cerebral blood flow
Affecting co-ordination and decision-making which could affect movement efficiency. Also reducing peak oxygen uptake by muscles and affecting the removal of metabolic waste from muscles. It appears that dehydration can also reduce muscular power and strength (Adams and Douglas, n.d.). - Increased body temperature
Resulting in vasodilation of blood vessels and increased blood flow to the skin for removal of heat. This redirection of blood flow further limits blood flow to working muscles. These effects are even more evident in hotter conditions. - Increased cardiovascular strain (via increased heart rate)
- Reduced blood volume leads to reduced stroke volume and cardiac output. This means, to maintain exercise output the heart must work harder. This leads to a higher heart rate which means the athlete will find maintaining their pace more difficult as they will have to work at a higher percentage of maximal effort.
- Increased glycogenolysis (break down and use of glycogen for fuel)
Likely due to increased intensity of effort and reduced peak oxygen uptake (O2 is required to metabolise fat). From a performance perspective, exhausting carbohydrate stores faster will lead to earlier onset of fatigue.
A number of perceptual dehydration effects are also evident during endurance exercise including:
- Stimulation of the thirst mechanism
- A lowering in mood
- Increased perception of effort – in other words a higher perceived exertion rate.
These physiological and perceptual effects are thought to act in combination to produce a level of discomfort that will distract from the task at hand and thereby compromise performance (James et al, 2019). The diagram below shows the interrelation of these effects and how they lead to impaired endurance performance. (Note: “hypohydration= dehydration)
It also appears that the effects of dehydration on endurance performance may be somewhat less in sports where the athlete does not need to carry their body weight (e.g., cycling). A meta-analysis performed by Goulet (2012) concluded that while the effects described above were present in lab-based constant intensity endurance experiments, the same decrement in performance was not apparent when cycling in real world conditions (i.e., when cycling outside). Goulet (2012) suggests that while performance deficits were seen at around 2% body mass loss during indoor fixed intensity experiments, evidence from multiple studies indicated that under real world conditions, exercise induced dehydration of up to 4% of bodyweight was unlikely to impair endurance performance in cycling. The authors concluded that this was because athletes were able to freely choose to manipulate their work rates, compared to lab-based experiments where they were asked to maintain a specific pace. These findings, while interesting, have limitations as the very idea that athletes can choose to manipulate their work rates, indicates athletes may not have been working to their maximal potential.
What is clear, is that endurance athletes will almost certainly become dehydrated during training and competition, so they need to build a tolerance for exercising while dehydrated. It is also clear that it is possible to achieve fast endurance times when affected by significant dehydration as in many modes of exercise, sweat losses are considerable and drinking opportunities are sparse. However, a targeted drinking plan that somewhat offsets fluid losses appears to be the most prudent approach.
Intermittent activity (i.e. sports)
There is clear evidence that dehydration also impairs performance in sports. This is particularly evident in those sports requiring longer running distances, for example, football, hockey, rugby, etc.
Physiological issues arise for similar reasons as in endurance exercise (diminished blood flow, elevated temperature, etc.). Yet, cognitive impacts might hold even greater significance in sports due to the requirement for decision-making, hand-eye coordination, motor skills, and reflexes—all of which are prone to decline when dehydration is present (Wittbrodt and Millard-Stafford, 2018).
How much sweat do sporting athletes lose?
Barnes et al (2019) included 1022 team/skill sports athletes in their sweat loss study and reported an average sweat loss of 1.10L per hour (+/- 0.58L per hour). The sports included in this study were AFL, baseball, basketball, American Football, cricket, ice hockey, soccer, tennis, and volleyball. To show how different sports were compared, they presented the data from the 4 sports where the number of athletes in the study exceeded 100 subjects.
The following table presents the data on sweat loss per hour and the corresponding percentage of body mass lost per hour in various sports. These values are based on measurements taken from athletic participants, with the averages noted for each sport.
SPORT | SWEAT LOSS PER HOUR (L) | BODY MASS LOST PER HOUR (%) |
---|---|---|
Baseball | 0.83 (+/- 0.34) | 0.9 |
Basketball | 0.95 (+/- 0.42) | 1 |
American Football | 1.51 (+/- 0.7) | 1.35 |
Soccer | 0.94 (+/- 0.38) | 1.4 |
The authors also reported that the sweat rates for adult men were also consistently higher than for women and youth athletes included in the study. Overall, male athletes lost 23% more sweat than female athletes and 9% more than youth athletes. Male athletes also lost 32% more sodium in sweat than females and 13% more than youth athletes, In summary, it would appear that sporting athletes lose on average less sweat than endurance athletes per hour of work and have more opportunity to re-hydrate during trainings and competition, meaning the effects of dehydration can be more easily mediated once the sweat rates of athletes are known.
As an example, if a soccer player loses on average 1.4L-2L of sweat a game, they would need to drink only around 500ml to 1L of fluid during a game to prevent them losing 2% of their bodyweight during a game. This is essentially the consumption of a bottle of water, which is relatively simple across 90 minutes.
Power athletes and resistance training populations
Adams and Douglas (n.d.) performed a literature review on the influence of hydration on muscular power and strength and concluded that while evidence from studies in this area was somewhat conflicting (due to variations in study methodology), dehydration did appear to reduce muscular power and strength. It was suggested that deficits in muscular power and strength as a result of dehydration were mainly due to factirs affecting the neuromuscular system that limited optimal fibre recruitment. These authors concluded that the available literature suggested that a 3-4% reduction in body mass from dehydration appeared to reduce muscular power output by about 3% (Adams and Douglas). While it was suggested that body mass losses of 3-4% were possible in longer training sessions and in higher temperatures, it was likely that they would only occur if an athlete was dehydrated coming into training.
How much sweat do power and resistance training athletes lose?
Barnes et al (2019) collected sweat data from individual power athletes (wrestlers, power lifters, and hammer/shotput throwers) who recorded sweat rates of 0.88L per hour of training (+/- 0.25L per hour).
These authors also collected sweat data from non-competitive gym going athletes and reported average sweat losses of 1.05L per hour of training (+/- 0.5L per hour).
Once again, these athletes have more than ample opportunities to consume fluid during trainings to attenuate fluid losses, with many resistance training athletes actually consuming more fluid than they lost during sessions.
Environmental Factors that Influence Fluid loss
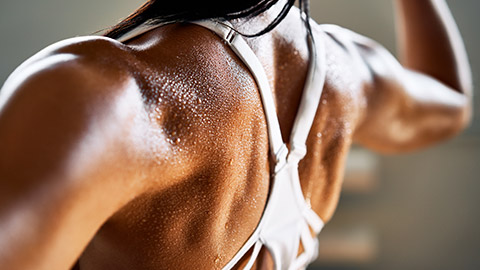
External factors like environmental temperature and humidity can have a large impact on our fluid losses during exercise. Before we dive into their effects, it is important to have a clear understanding of heat transfer systems in the body, so you can better understand how these external factors impact on them.
In order to function optimally, the body likes to maintain a homeostatic internal temperature of around 37 degrees Celsius. It does this by producing heat to counter the heat we lose via various processes.
Internal body heat is generated and maintained predominantly via metabolism. That is, when calories are burned by cells, a by-product of this cellular metabolism is heat. When we are cold, our body will attempt to maintain our temperature by increasing our metabolism. The easiest way to do this is to move.
During exercise, our muscles increase their metabolic rate, thus increasing body temperature. The harder we work, the more calories we metabolise and the more heat we generate. Men generally sweat more than women during exercise due to larger muscle mass, which (when working) creates larger calorie metabolism and more heat.
If we become too hot internally, our body will begin to focus on off-loading heat. During exercise, this is usually achieved through sweating. But sweating is not the only way in which we lose heat. Heat loss from the body occurs in 4 primary ways:
Note: Heat transfer works both ways, for example we can absorb radiation (e.g. from the sun, or a heater) or by holding warm objects (e.g. hot water bottle).
This following video explains how the body maintains temperature:
How temperature affects performance
Heat loss mechanisms are dependent on the difference in temperature between the skin and the environment. While internal body temperature sits at around 37 degrees Celsius, skin temperature varies depending on where in the body it is located. The image below shows the ratio of typical skin temperatures according to body location and ambient temperature. As you can see, the head and back of the neck hold the highest skin temperatures followed by parts of the trunk. The lowest skin temperatures are in the extremities.
Skin location | Cold (15 ºC) | Room (27 ºC) | Hot (47 ºC) |
---|---|---|---|
A: Forehead | 31.7 | 35.2 | 37 |
B: Back of neck | 31.2 | 35.1 | 36.1 |
C: Chest | 30.1 | 34.4 | 35.8 |
D: Upper back | 30.7 | 34.4 | 36.3 |
E: Lower back | 29.2 | 33.7 | 36.6 |
F: Upper abdomen | 29.0 | 34.8 | 35.7 |
G: Lower abdomen | 29.2 | 33.2 | 36.2 |
H: Tricep | 28.0 | 34.0 | 36.6 |
I: Forearm | 26.9 | 33.8 | 37.0 |
J: Hand | 23.7 | 33.8 | 36.7 |
K: Hip | 26.5 | 32.2 | 36.8 |
L: Side thigh | 27.3 | 33.0 | 36.5 |
M: Front thigh | 29.4 | 33.7 | 36.7 |
N: Back thigh | 25.5 | 32.2 | 36.0 |
O: Calf | 25.1 | 31.6 | 35.9 |
P: Foot | 23.2 | 30.4 | 36.2 |
The main issues that arise from exercising in hot conditions appear to be:
- Rising core temperatures.
- An increase in energy used to remove additional heat.
- A greater reduction in blood volume due to increased sweating leading to reduced aerobic capacity.
- A reliance on glycogen (carbs) as a fuel source.
- Increase lactate accumulation leading to fatigue.
- Greater perceived exertion rates.
These issues are all explained in greater detail below:
When exercising in temperatures higher than skin temperature, the body gains additional heat from the atmosphere via radiation. This means the body has to sweat more in an attempt to maintain internal temperature at 37 degrees. As the temperature rises, more and more energy is spent on removing excess heat through evaporation (accumulating from both the metabolic action of muscle and heat gained from the environment). This additional effort combined with reducing blood volume (through sweat loss) increases the intensity with which an athlete must work to maintain their physical work. The result of this is a shift in energy production from aerobic to anaerobic mechanisms, which leads to more rapid consumption of muscle glycogen stores and ultimately a reduction in performance output (Intermountain Health Care, 2017). Multiple studies have shown an increase in muscle glycogen use and subsequent decrease in fat use as a fuel during exercise in heat. This growing reliance on glycogen as fuel for aerobic exercise also resulted in higher blood lactate levels which could lead to early onset of fatigue (Sawka et al, 1993).
The hotter the ambient temperature, the more we rely on sweat as the mechanism for heat removal and the more fluid we lose. The image below shows the changes to how heat is removed from the body while exercising in different temperatures. This data was collected from a cyclist who maintained a constant cycling intensity for 1 hour progressing through incremental ambient temperature increase in a heat chamber. What the data shows is that total heat losses stay relatively constant in line with the athlete’s metabolic rate, but that the way in which the athlete removed the heat shifted dramatically from “sensible heat loss” (radiation) at lower temperatures, to an almost complete reliance on “insensible heat loss” (sweating) at higher temperatures (Sawka et al, 1993). At 10 degrees Celsius, 70% of heat loss was through radiation. When the ambient temperature reached skin temperature (in the 25 degrees Celsius range) sweating accounted for almost all of the heat loss. The authors also found that when ambient temperature rose above skin temperature (i.e. into the 30-degree Celsius range), the body started to gain heat from the atmosphere and body temperature increased.
This increase in core temperature when ambient temperature rises above skin temperature can be seen in the image below which shows data from a cyclist who performed three separate workouts at different intensities in progressively increasing heat. You can clearly see that regardless of the exercise intensity performed, core temperature rose noticeably once ambient temperature rose above 25 degrees Celsius
Maughan and Shirreffs (2004) suggest the impact of dehydration on performance is more noticeable in hotter environments than in cooler. They suggest that the same level of dehydration may be more tolerable in cool climates, which further indicates that increase consumption of fluid is necessary as ambient temperature increases. This rise in body temperature leads to increased displacement of blood to the skin (in an attempt to cool the body) reducing venous return and cardiac output. This leads to reduced ability for athletes to reach maximal aerobic metabolic rate as oxygen delivery and uptake by muscle is impaired (Sawka et al,1993).
Interestingly, even when the effects of dehydration are mediated through fluid loss, athletes tend to perceive exercise as harder when it is performed in higher ambient temperatures even though key physiological measures of heart rate and core temperature haven’t changed (Douglas, 2019).
How humidity affects performance
Ever wondered why your washing won’t dry completely on the clothesline when it’s hot and muggy? That’s due to humidity. The term “humidity” refers to the water content of the air. Low humidity means there is little water vapour in the air, so low humidity allows for greater evaporation of fluid from surfaces, your skin (and of course your clothes). Essentially the air is not saturated with water, so is willing to accept more.
High humidity means the air is already saturated with water vapour. As a result, the air is less willing to accept moisture. In terms of sweating to maintain a constant internal temperature during exercise, humidity poses an additional problem to heat. In order for sweat to cool the body, it must evaporate. It is the chemical reaction of turning liquid (sweat) into gas (air) that creates the cooling effect. If sweat remains on the skin, or drips off the skin, the cooling effect is lost, along with the fluid. This means an athlete not only loses vital fluid, but also receives no cooling effect from this loss. This means when the humidity of the environment is high, the rate at which sweat evaporates from the skin is lower than it would be under dry conditions. This is likely to result in a marked rise in core temperature despite ongoing sweat losses (Maughan, Otani and Watson, 2012).
While elevated core temperature, increased sweat rates and decreased sweat evaporation have all be observed in humid conditions, there has been less research on the effect that this has on exercise performance. Maughan, Otani and Watson (2012) compared the effects of different humidity levels on incremental cycle performance. Temperature was maintained at 30 degrees Celsius, while the different time to exhaustion trials were conducted at 24%, 40%, 60% and 80% humidity levels. The authors concluded that cycling performance was progressively impaired as the relative humidity increased. Performance decrements were 12% from 24-48% humidity and even larger deficits occurred as humidity increased further. The authors also noted that even though the subjects core temperature rose to the same levels in all trials (around 39 degrees Celsius) it rose much earlier in the higher humidities (Maughan, Otani and Watson, 2012).
While the reasons why high humidity reduces exercise performance are believed to be related to additional sweat losses and higher core temperatures, there is also strong beliefs that the discomfort of exercising in humid conditions plays a part. One theory is that because sweat doesn’t evaporate and cool the skin, skin temperatures remain high. Multiple studies have found a correlation between skin temperature and comfort and Maughan, Otani and Watson (2012) believe that the discomfort of high skin temperatures is a likely factor in performance decreases.
Adaptation to training in heat and humidity
The good news is it appears athletes can acclimatise to higher temperatures and humidity. The most common way of acclimatising is through repeated exposure to training in higher temperatures and humidities. Ideally this involves spending a period of time in a hotter/humid climate, but acclimatisation has also been shown through use of a heat chamber for up to 1-2 hours a day. Tyler et al (2016) performed a meta-analysis on a range of heat acclimatisation protocols and concluded the following:
- Heat acclimatisation had a moderately beneficial effect on exercise capacity and performance in the heat irrespective of regimen; however, longer regimens (over 14 days) were more effective than shorter approaches.
- Heat acclimatisation had moderate-to-large beneficial effects on lowering core body temperature before and during exercise, maintaining cardiovascular stability, and improving heat-loss pathways.
- Heat acclimatisation may reduce oxygen consumption during exercise in heat, improve glycogen sparing, increase the power output at lactate threshold, reduce lactate concentrations during exercise and improve perceived ratings of exertion.
Tyler et al (2016) provided a list of guidelines athletes who wish to try and acclimatise for heat and humidity:
- Athletes should spend as much time as possible exposed to high ambient temperatures to maximise performance, physiological, and perceptual adaptations. Ideally, preparation for competition in hot temperatures should start at least 14 days prior to the event. Some adaptation does occur withing the first few days training in heat, and adaptation appears to be more or less complete within 7-14 days.
- Temperatures of between 30 and 35 degrees Celsius are considered optimal for acclimatisation.
- Higher-intensity exercise may offer a more rapid heat acclimatisation stimulus than lower-intensity exercise and so could be considered when time constraints prevent a longer heat acclimatisation regimen (benefits were seen in as little as 6 days in some studies).
- Where possible, active, rather than passive heat acclimatisation should be undertaken - i.e. not just living in heat, but training in heat. 60 to 100 minutes of moderately strenuous activity in the heat appears to be optimal for acclimatisation. Intermittent exercise in heat is likely to be equally effective as continuous exercise.
- Athetes should aim to reduce body temperature as quickly as possible following acclimatisation training. This can be achieved by moving into air-conditioned environments or by use of a cool shower. Cool fluids should be consumed at regular intervals.
- The ambient temperatures used should mimic the conditions which will be encountered. Due to reductions in work capacity in the heat the thermal stress may need to be progressively increased to ensure deconditioning doesn’t occur. This means some trainings may be best done in cooler conditions to maintain fitness condition.
- Exercise intensity during the heat acclimatisation should be monitored and progressively increased to ensure that overloading and adaptation continue to occur. HR may be the most practical way to ensure an appropriate magnitude of physiological strain during acclimatisation when the measurement of core body temperature is impractical. Training intensity will have to be reduced for the first few days in higher temper
- Where possible, consecutive days of heat acclimatisation should be undertaken; however, if this is not possible heat exposure every other, or every third, day can still induce beneficial adaptations.
- If travelling to a hot location is not an option, acclimation can be completed by exercising in artificial heat (e.g. a heat chamber or hot room). Here is an example of this type of acclimation in action:
Athletes and trainers should also be aware that acclimatisation does not result in less fluid requirement. In fact, the opposite is true. Heat acclimatisation actually increases the requirement for fluid replacement due to an advanced sweating response. Athletes therefore not only have to drink more in heat, but also have to drink more as they become acclimatised as they begin to sweat even more (Maughan and Shirreffs, 2004)
Other strategies that can be used to combat the effects of exercise in high heat
While acclimatisation to heat appears to be the best way to prepare for competing in hot and humid conditions, a number of other strategies have been developed to aid performance in hot conditions. These are mainly used by endurance athletes but could easily be adopted by team sport and other individual athletes also.
Pre-cooling strategies | In simple terms this involves cooling of the torso prior to starting an exercise bout in the heat. The most common ways of doing this appear to be ice or cool water immersions, the wearing of a cooling vest and the consumption of an ice slushy in the pre-exercise period. |
---|---|
Per-cooling strategies | This involves the application of additional cooling strategies during exercise competition. The most common approaches used appear to be wearing cooling vests, application of cooling packs and consumption of an ice slushy. |
A meta-analysis performed by Bongers et al (2014) reviewed 28 studies involving 323 participants that had examined pre and per-cooling strategies and concluded the following:
- The overall effect of pre and per-cooling strategies on exercise performance was a 6.7% improvement.
- Per-cooling strategies had a higher performance effect than pre-cooling (particularly in longer events). This was thought to be because the effects of pre-cooling strategies appear to wear off after around 20-25 minutes of activity in heat.
- Both strategies resulted in lower finishing core temperatures (38.9 degrees Celsius vs 39.1 degrees Celsius in control groups).
- Pre-cooling seemed to work better if a combination of strategies were used including use of cooling-vests and consumption of ice-slushies. The use of cooling vest was found to be the most effective single approach for pre-cooling. Cooling vests were also the most effective per-cooling strategy. While this may be difficult to implement during activity, the use of cooling vests during substitutions or breaks in play (quarter and half-time) may be beneficial for sporting athletes.
- The overall theory behind how pre and per-cooling strategies work appears to be the increase in body heat storage capacity they facilitate. This means athletes can produce more heat before having to terminate exercise or lower their intensity, which translates into performance enhancement.
- While the torso appeared to be the most important area of the body to cool, there was evidence that the higher the area of the body receiving cooling, the better the outcome.
The following is an example of high-performance athletes using a cooling vest that might achieve both pre and per-cooling effects.
So, we now know a lot about fluid! We have established what to drink, how to drink it, why we need it, how we lose it and the factors that affect how much we lose. We have even learned about some strategies we can look to employ if our athletes are going to be competing in very hot conditions.
Before we go onto to the current recommendations for drinking during and after exercise, a key point must be emphasised!
The simplest thing we can do to ensure we get the most out of trainings for ourselves and our clients is to ensure we begin any exercise in a hydrated state. This of course can be monitored through checking urine colour and weight (as previously discussed). Going into any but of exercise with a less than optimal hydration status means you will reach impairment levels sooner during the session.
Here are the current pre-exercise drinking recommendations according to the National Strength and Conditioning Association (NSCA, 2019).
- Beginning exercise fully hydrated and with normal electrolyte levels is usually easily achieved by drinking water at regular intervals throughout the day and by consuming high water content foods such as fruits and vegetables. If at least 8 to 12 hours have passed since the last exercise session and fluid consumption has been adequate, then individuals should be close to an optimal hydration state. On the other hand, for someone who has lost a significant amount of fluid during exercise and has not replenished with fluids and electrolytes in the amounts needed to establish hydration levels, an aggressive pre-exercise hydration protocol should be adopted.
- Starting at least 4 hours before exercise, athletes should consume approximately 5 to 7 ml fluid per kilogram body weight. This would equate to between 375 and 525ml of water for a 75kg athlete. This should be consumed in a slow and controlled fashion (sipping over time).
- If at 2 hours before exercise, the athlete has not urinated or urine is dark in nature, athletes should begin to slowly consume approximately 3 to 5 ml/kg body weight. Consuming sodium-rich foods at this time can help stimulate thirst and retain fluids. If sodium is consumed in a beverage, the recommended amount is 460-1,150mg per litre of fluid (around quarter of a tsp of salt). Sodium consumption in the pre-exercise window can also assist with retention of water helping to ensure optimal hydration for the start of the exercise bout (Shirreffs and Sawka, 2011).
- If palatability (taste) is a limiting factor to consumption of pre-exercise fluid, water can be lightly sweetened with the addition of fruit juice. Avoid caffeinated or alcoholic beverages.
Predicting hydration requirements during exercise
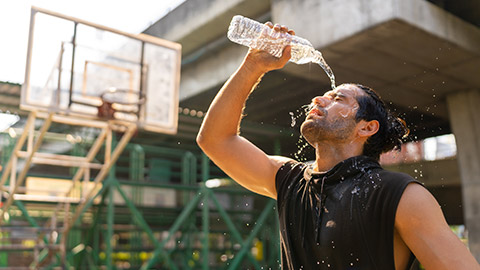
The goal of drinking during exercise is to prevent excessive dehydration (greater than 2% of body weight lost in sweat) and excessive changes in electrolyte balance. This is best achieved by replacing at least 80% of sweat losses during exercise. The magnitude of sweat losses incurred during exercise is affected by a number of variables including:
- The intensity, duration and type of the exercise
- The ambient temperature and humidity of the exercise environment
- Wind (both as a means of increasing intensity if working into it, and in its effect on heat transfer through convection)
- Clothing or equipment requirements (the more skin exposed the greater the cooling effect)
The most important of these factors appear to be the intensity and duration of activity (which affects metabolic heat production) and the environmental temperature and humidity which can lead to additional heat gain and reduced effectiveness of sweating (Sawka et al, 2005).
Acute exercise sweat losses commonly fall within the range of 1-2L per hour for team and individual endurance sports. There are considerable variations in fluid requirement for different sports and also different positions within a sport. These are typically aligned with work rates and running distances (Sawka et al, 2005).
Maximal gastric emptying rates appear to be around 1-1.5L of fluid an hour. This means it is possible to match fluid intake with sweat losses, except for in exercise in hot and humid conditions where fluid losses can easily exceed 2L/hour (Sawka et al, 2005).
Most studies indicate only half of sweat loss volumes are typically replaced during exercise by most athletes which generally results in moderate dehydration of 1-2% of body weight lost in team sport athletes and 1-4% of body weight lost in competitive endurance athletes (Sawka et al, 2005). The greater fluid deficits shown in endurance athletes is often down to fluid availability during events and trainings.
What the information above shows us is that most athletes are operating in a dehydrated state for at least some of their trainings or competitions and maybe even the whole time if they enter the exercise bout in a dehydrated state. Given that a body fluid loss resulting in 2% of body weight is strongly correlated with impaired performance, it would be in an athlete’s best interest to limit body weight losses to less than 2% to enable them to continue to perform at a high level.
But here is where the issues lies. There is no formula for what an ideal amount of fluid consumption is. Which means any general recommendations for fluid intake around exercise are somewhat arbitrary. This is because there are too many variables associated with exercise (and individuals) to suggest an amount of fluid that will satisfy fluid requirements for any exercise bout.
Weighing clients before and after exercise
The closest we can get to individualising athlete fluid requirements is by weighing athletes before and after exercise. This will give a general idea of the amount of fluid lost during sessions of different intensities and durations. If notes are also taken around fluid losses in different temperatures and humidities, an even better prediction of fluid requirements for particular sessions can be established. This is obviously a time consuming and tedious task, but even weighing clients before and after exercise in the gym can give indications of fluid general fluid losses and at the very least will let an athlete/client know how much fluid they need to replace post training to fully rehydrate. Here are the steps to collecting and using pre and post exercise weighing for better fluid management:
- Ensure the client is hydrated prior to weighing by following the pre-exercise fluid guidelines already discussed (urine is clear).
- Weigh the client in light clothing (additional layers removed) - note pre-exercise weight
- Perform the exercise session keeping note of all fluids consumed. Encourage the client to drink regularly.
- Weigh the client post training. Wipe off all sweat from skin and remove sweat logged clothing if possible – record post exercise weight.
Any weight deficit from pre-to post weigh in along with the amount of fluid consumed during , represents the level of dehydration the session caused. If the session was an hour, you now have a sweat rate per hour figure. If the session was over an hour, simply divide the total fluid loss by the duration of the session, the multiply that figure by 60 to get your sweat rate per hour figure.
For example, if an athlete weighed 70kg at the start of a 90-minute session and 68.8kg at the end of the session, they have lost 1.2kg in fluid. If they drank 600ml during the session, their total fluid loss is 1.2L + 600ml = 1.8L (or 1800ml). The session duration was 90 minutes, so 1800ml/ 90 = 20ml per min. Multiply this by 60 (minutes) and their sweat rate per hour is 1200ml.
In this instance, a considerable weight deficit has occurred. This athlete can now use the calculated sweat sweat-rate to create a clear fluid consumption plan for the next session. They know how much sweat they lost in an hour (1200ml), so can attempt to consume this amount by breaking it up into regular consumption intervals. In this case, the athlete would have to try and consume 300ml every 15 minutes of exercise.
Remember the aim is to match fluid losses as closely as you can, however for clients who struggle to tolerate that level of fluid consumption, aiming for 80% replacement is a strategy employed by many trainers. This appears to be sufficient to prevent body weight losses of up to 2% in most instances. For the client example above this would equate to 240ml (or 1 cup) every 15 minutes of training.
General fluid consumption guidelines during exercise
While there are no fool proof recommendations for fluid intake during exercise, scientific data from monitoring athlete fluid losses across a wide range of activities in varying environments has allowed the experts to come up with a set of recommendations that can be used as a starting point for fluid consumption during exercise. The suggestion is, that rather than waiting for the results of pre and post weigh ins, these figures can be used to off-set fuid losses during sessions, and then adjusted once weight information has been compiled. These recommendations come from the NSCA (2019):
Hydration strategies for different sports
- Try to drink small amounts of fluid (150-300 mL depending on ambient temperature) every 15 to 20 minutes of exercise. This volume can be varied according to individual sweat rate (this can only be achieved through weighing). Core temperature, heart rate, and perceived effort remain lowest when fluid replacement comes closest to matching the rate of sweat loss
- During sport/exercise lasting less than 60 minutes, water alone is sufficient for fluid replacement provided carbohydrate and sodium have been consume in a pre-exercise meal. If not (as in early morning workouts) a sports drink containing no more than 4-8% carbohydrate per 100ml and some sodium could be beneficial as it aids absorption. Commercial sports drinks would be fine. Avoid drinks higher in sugar as these delays gastric emptying and can cause gastro-intestinal upsets.
- During prolonged exercise lasting longer than 60 minutes, commercially available carbohydrate electrolyte beverages should be considered to provide an exogenous carbohydrate source to sustain carbohydrate oxidation and endurance performance.
- Electrolyte supplementation is generally not necessary because dietary intake is adequate to offset electrolytes lost in sweat and urine; however, during initial days of hot-weather training, when meals are not calorically adequate, or for intense exercise that has gone beyond 60 minutes duration, supplemental sodium intake may be indicated to sustain sodium balance. This is particularly necessary for heavy sweaters and those that sweat high sodium concentrations (i.e. if you notice sweat markings on clothing or skin). Most commercial sports drinks contain 35mg of sodium per 100ml which is adequate for most exercise scenarios, although may not be enough to off-set electrolyte losses in sweat in higher temperatures or ultra-endurance events. In these conditions 50mg of sodium per 100ml is suggested.
Additional notes for certain types of exercise
Let's find out more into some specialised considerations for certain types of exercise.
Endurance and ultra-endurance
It appears that water may not the optimum fluid for ingestion during endurance exercise, and there is compelling evidence that drinks containing added substrate and electrolytes are more effective in improving performance. Increasing the carbohydrate content of a drink will increase the amount of fuel available but will tend to decrease the rate at which water can be made available (Shirreffs, 2009).
Where provision of water is the priority, the carbohydrate content of a drink and its total osmolality should be low, thus restricting the rate at which substrate is provided. This is why water is recommended for activities lasting less than 60 minutes. It also means, that during the earlier parts of endurance training or competition, fluids with lower amounts of carbohydrate are recommended (2.5-4%/100ml). As activity continues and a reduction in total body glycogen occurs, the sugar content of fluid solutions should increase to around 6-8% per 100mls and the inclusion of sodium becomes more important as the exercise duration continues (Shirreffs, 2009). The recommended amount of sodium to include in fluid during longer duration exercise is 0.5 to 0.7 g/L of fluid (Von Duvillard et al. 2004). More recent research has also indicated that women’s blood sodium levels are typically lower than men’s during ultra-endurance events meaning the inclusion of sodium may be even more important for females (White, n.d.).
One further consideration for ultra-endurance athletes is that they should not attempt to ingest all their carbohydrate and sodium requirements in fluids. In races lasting over 4 hours duration, the consumption of some solid food is recommended to avoid gastro-intestinal distress (White, n.d.).
Recent research also suggests that ultra-endurance athletes competing at relatively low intensities, or smaller athletes with relatively low metabolic sweat rates may not require the volumes suggested in the drinking recommendation volumes discussed above. Female ultra-endurance athletes in particular may have lower fluid volume requirements due to having lower sweat rates, being smaller and having smaller fluid compartments and because they take longer than men to complete events (White, n.d.). The exact volumes these athletes require can of course be calculated from pre and post event weighing.
Resistance training
Resistance training total calorie outputs are generally much lower than for cardio workouts. This means sweat rates are often lower also. This, plus the fact that fluid consumption is readily available and rest periods plentiful during resistance training, means that drinking plans are not as vital for resistance training sessions. As long as a client starts the session hydrated, and is well fuelled prior to the session, regular consumption of water in rest periods will ensure adequate hydration. In fact, many resistance-trained athletes finish a session heavier than they went into the session (not necessarily a good thing by the way).
Fluid consumption guidelines for after exercise
Athletes should aim to replace 1.5 x the amount of body weight lost during exercise. The only way to accurately determine this amount is to weigh athletes before and after training, games or competitions. The additional fluid is needed to compensate for ongoing sweating and urine loss. It is recommended that these fluids should contain some electrolytes or should be consumed alongside foods containing salt (Sherriffs et al, 2004). This is particularly important when another bout of exercise will follow within a relatively short period of time (for example in tournament settings) as sodium improves both the absorption and retention of water. It also prevents the dilution of body electrolytes (as can happen when water is drunk too quickly). Fluid should be consumed slowly and regularly to optimise absorption and fluids containing caffeine or alcohol should be avoided. Replacing lost fluids within no more than 4 hours post training not only restores your hydration status but also has been shown to improve recovery from exercise and reduce post-exercise fatigue (Sayer, 2022
Sayer (2022) suggests the following timelines to achieve full re-hydration following different levels of dehydration:
Mild dehydration | Around 45 minutes |
---|---|
Moderate dehydration | Replace fluids over the same time as they were lost. For example, if you lost the fluids over 90 minutes of exercise, replace lost fluids over the same time period. |
Severe dehydration | Often takes 24 hours or more and will likely require IV fluid replacement. |
The ideal rate of fluid replacement to ensure optimal fluid absorption and full re-hydration appears to be 1L of fluid an hour (Sayer, 2022).
Professional athlete environments
Professional athlete environments are well aware of the need to monitor athlete sweat rates.
Check out the following videos that shows how both sports teams and individual athletes measure their fluid requirements to ensure they can perform optimally.
Note: You'll observe that the events are sponsored by Gatorade. Rest assured, the content presented in these videos is grounded in scientific evidence.
Right, time to apply what you have learned. Head to your assessment for an assessment guide video and instructions on submitting your assessments.
The assessment guide video explains your assessment task, which requires you to use the information you have learned on this topic to help a case study client.
This assessment will require you to apply the knowledge you have learned and practised by completing a case study relating to hydration strategies for a travelling sports team who will be competing in very hot and humid conditions.
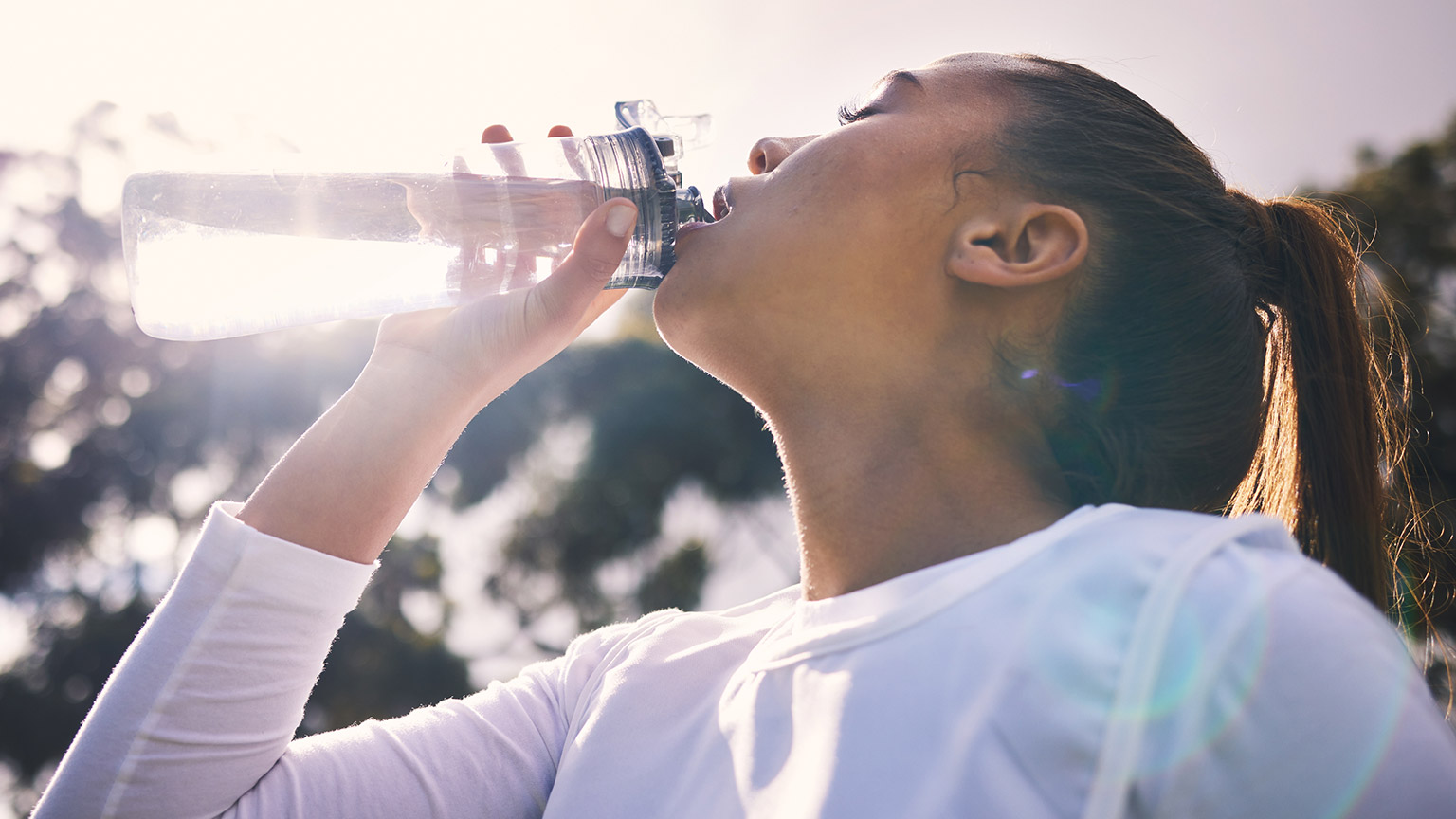