In this topic, we explore the structure and function of the nervous system. The nervous system is responsible for tasks that help maintain homeostasis, so there is a lot of detail. Use the activities to check your understanding frequently.
The nervous system does not maintain homeostasis alone. It works with other systems to maintain a safe functioning working environment for the human body.
Think of the nervous system as the control centre. It is the receiver of all messages from the other 10 body systems and is the brains of the operation. The nervous system:
- receives messages from the other body systems.
- translates them as to what, why, where and when attention is needed and how it will perform this action in the body.
- communicates this to other systems and lets them know what they need to do.
This is teamwork!
Understanding the nervous system and its components will help you understand how muscles work in terms of exercise and when working out and cooling down. This will equip you to be confident and knowledgeable while providing your clients a safe and valuable experience.
In this topic, you will learn:
- Nerve anatomy and physiology
- The nervous system and exercise
- Nervous system adaptations.
Structure and Function
The nervous system carries out numerous functions within the human body. It enables us to speak, smell, remember past information, make new memories, and learn. It provides signals which control body posture and movements, reflexes, and regulates our internal environment.
The nervous system is divided into 3 basic functions:
- Sensory input
- Integration (processing)
- Motor output
1. Sensory input
The sensory receptors detect stimuli. They detect information such as increased body temperature or external stimuli such as a cold breeze. This sensory information is carried to the brain and spinal cord for processing through the spinal and cranial nerves.
2. Integration
This is when the nervous system analyses the sensory input and makes a decision as to how and when to respond.
3. Motor output
The nervous system sends messages (also known as motor responses) that activate organs to move and/or the glands to secrete their substances.
What does the nervous system do?
The nervous system and the endocrine system work to control all activities within the human body. The nervous system quickly communicates messages between the brain and the body using nerve impulses (also known as action potentials).
The 4 main functions of the nervous system are:
- Control of the internal environment to achieve homeostasis
- Reflexes
- Memory and learning
- Voluntary control of movement
These functions are relevant for personal trainers to understand and be aware of when programming. Let's look at these functions in a bit more detail.
Control of the internal environment to achieve homeostasis
The nervous system carries out homeostasis to ensure internal conditions stay constant. This includes maintaining blood glucose levels, body temperature, and water levels.
Receptor cells detect changes in the environment (stimuli). The central nervous system (CNS) receives and processes the information and communicates to effector cells (e.g., muscles and glands) what to do to restore the body to optimal levels.
For example, when we exercise, we create heat. To maintain a relatively constant temperature, the nervous system sends messages to blood vessels to dilate, increasing blood flow to the skin and increasing sweating. This helps disperse the heat.
Reflexes
Reflex activity is when a stimulus leads to immediate action. The action is an inborn central nervous system activity, not involving consciousness.
A well-known example of this is the pupillary light reflex. If a light is flashed near one eye, the pupils of both eyes contract. Light is the stimulus; impulses reach the brain via the optic nerve, and the response is conveyed to the pupillary musculature by autonomic nerves that supply the eye.
Reflex functions protect us from injury. If you are jogging and accidentally roll your ankle, the stretch reflex instantly protects the ankle from breaking, limiting the injury to a sprain. The instant stretch in the muscles around the ankle is the stimulus. The CNS interprets this and sends a message to the muscles to tell them to contract and resist the stretch.
Memory and learning
As you learn, messages travel from one neuron to another over and over, creating connections, or pathways, in the brain. The first time you do something, it requires a lot of concentration and effort. But with practice, the connections (or pathways) are strengthened and the task or memory recall becomes easier. The pathway becomes established.
Repetition assists learning and memorisation and this can be applied to exercise. New movements, especially complex ones, take time for the nervous system to learn.
Voluntary control of movement
Every voluntary movement a person performs is under the direct control of the nervous system. The nervous system sends messages to the targetted body parts to move. If the movement has been repeated many times, the movement will be very efficient. E.g., walking. If the movement is new, it will be less efficient and may look awkward and ungainly. E.g., a person learning to squat for the first time.
Hierarchical structure
The nervous system has many divisions, each with its own distinct purpose. Here you can see the hierarchy of the nervous system and how the various divisions are organised:
What makes up the nervous system?
While many components make up the nervous system, the 3 general classifications of components are:
- The brain: The brain is the supercomputer of the body. It processes billions of pieces of information and decides how and what we should focus on, respond to, or ignore.
- The spinal cord: The spinal cord is the superhighway for communication. It carries messages between the peripheral nerves and the brain. This also stores vital reflex commands for flight or fight responses.
- The neurons (nerves): Neurons are the communication pathways between the body parts and the spinal cord. They also carry impulses up the spinal cord and to and from the different parts of the brain.
Let's take a look at each component in closer detail, starting with the brain.
1. The brain
The brain is made up of about 85 billion neurons! They equip the brain with the communication tools it needs to perform its function. The adult brain is anatomically subdivided into 4 major parts:
- Cerebrum
- Diencephalon
- Cerebellum
- Brain stem
It is important to have a comfortable understanding of their general functions, so let's explore each part.
The cerebrum
The cerebrum is the largest part of the brain. It is comprised of 4 lobes responsible for different processes and actions. For example, thinking, movement control, sensory perception, and sight. What are the specific functions of each lobe? Expand the accordion to find out.
The diencephalon
The diencephalon is where we find the hypothalamus, as well as other parts which are responsible for controlling the body's internal environment, such as temperature control, regulation of heart rate and breathing, hunger, sleep and wake cycles and more.
The cerebellum
The cerebellum sits behind the brain stem. It coordinates movements and helps maintain body awareness.
The brain stem
The brain stem continues from the spinal cord and essentially connects the spinal cord to the brain. The brain stem has regulatory functions involved in breathing and heart rate.
2. The spinal cord
The spinal cord is a long, thin, incredibly fragile tubular structure encased within the spinal column and surrounded by cerebrospinal fluid. It is composed of nervous tissue and tracts of nerve fibres that allow two-way conduction of nerve impulses, both sensory and motor (input and output). This is a key piece of knowledge to remember.
3. Neurons
The third classification for the components that make up the nervous system is the neurons (nerves). Nerve anatomy is a large and complex area so we will look at this next.
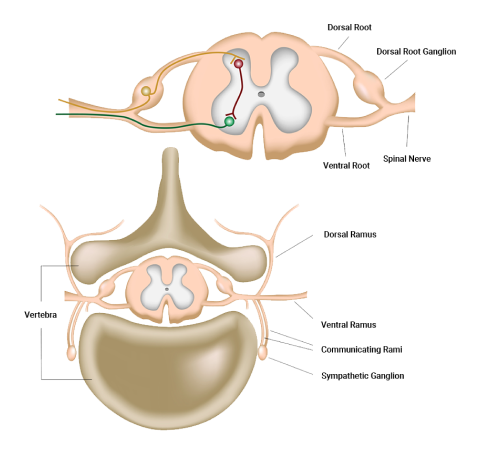
Nerve Anatomy
Neurons are the individual pathways that carry electrical impulses around the human body. Nerves are bundles of neurons all heading in the same direction, with neurons branching off them at points to reach their intended destinations.
Functional classification of neurons
Neurons are responsible for producing complex networks and connections between:
- the brain and the spinal cord.
- the rest of the body and the brain and spinal cord.
These lines of communication are composed of highly specialised cells capable of transmitting highly complex information at great speeds. There are 3 main types of neurons. They tag team each other in the journey of signal transmission.
- Sensory neuron
- Interneuron
- Motor neuron
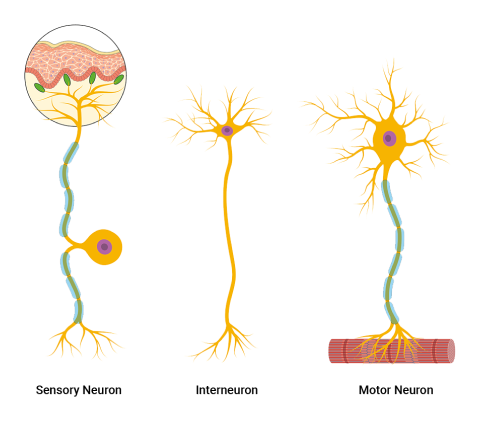
Sensory neurons | Sensory neurons 'sense' information and provide the link between sensory receptors and the spinal cord. They transport electrical impulses to the spinal cord, where the impulses are passed to interneurons. |
---|---|
Interneurons | Interneurons are found only within the spinal cord and brain (CNS) and are situated between sensory and motor neurons. They process incoming sensory information received from sensory neurons and then activate the related motor neuron. They carry impulses up to the brain for processing and decision-making. 90% of all neurons in the body are interneurons. |
Motor neurons | Motor neurons carry impulses that exit the spinal cord away from the CNS and are directed towards the effectors of the body, such as muscles, organs, and glands to cause action. |
Anatomy of a neuron
In reality, all neuron types look slightly different but contain the same key components. The picture below shows a generalised version of a neuron.
If you wish to learn more about the parts of a neuron, expand the accordion.
The control centre of the neuron is its cell body (also called the soma).
It has a centrally located nucleus which acts as the brain of the neuron.
The cell body usually gives rise to a few thick processes (sticky out bits) that branch into nerve fibres known as dendrites.
They house numerous sensory receptors for neurotransmitters and are the primary sites for receiving signals from other neurons.
The more dendrites a neuron has, the more information it can receive from other cells to incorporate into its decision-making.
All axons of the PNS (peripheral nervous system) have a sheath of Schwann cells around them. Each Schwann cell repeatedly spirals around the axon, putting down as many as 100 layers.
These layers form the myelin sheath. Think of the myelin sheath as similar to plastic around an electric cord. The myelin helps fully envelope the axon of the neuron to ensure there are no 'leaks' in information and to help speed the transmission of signals.
Myelinated nerve fibres conduct impulses faster than unmyelinated nerve fibres.
The axon is cylindrical and relatively un-branched for most of its length. It allows rapid transmission of nerve impulses from the cell body.
Axons may range from a few millimetres to more than a metre in length.
An axon typically connects with another muscle fibre or gland cell via its 'axon terminals'.
At the distal end, axons usually break into a complex network of fine branches. Each branch ends in a little swelling called a 'synaptic knob/bulb'.
Each knob forms a junction with a muscle cell, gland cell, or another neuron. The point where communication occurs between two neurons or a neuron and an effector cell is called a Synapse.
Nerve Impulse
The currency of the nervous system is electrical impulses. These are also known as Action Potentials (AP). These electrical impulses have a voltage.
Nerve pathway
Almost every nerve impulse follows the same pathway, starting from a sensory receptor and ending at the effector muscles.
- Sensory receptors notice a change in the internal, or external, environment and send impulses via sensory neurons to the central nervous system (brain and spinal cord) for processing.
- Interneurons in the brain or spinal cord (CNS) process these impulses and decide to act, store the information, or discard it.
- If action is warranted, the CNS sends impulses down the spinal cord via interrnuerons, and out using motor neurons, to the body's effectors to cause action (for example, to the muscles, glands, organs).
The image above shows the pathway of a nerve impulse. Sensory receptors notice pain from the stimulus (the cactus) and an impulse travels to the spine via sensory neurons. Once at the spine, interneurons travel up the spine to the brain where the sensation is relayed. The impulse travels back down the spinal cord via interneurons and out using motor neurons to the muscles where it activates the muscle fibres to perform a task - in this case, to move the hand away from the stimulus.
The synapse
A synapse is the site of communication between a neuron and another neuron or a neuron and an effector cell.
These meeting points allow impulses to be passed between the different neurons. The transmission of these electrical impulses contains a few steps and is somewhat complex at first, so let's break it down.
The essential components of a synapse are:
- The pre-synaptic terminal: This is the synaptic end bulb (or knob) of the first neuron. It is before the synapse. It contains neurotransmitters packaged into 'vesicles'. Neurotransmitters are chemical couriers that are vital in generating new impulses on the other side of the synaptic cleft.
- The synaptic cleft: A small gap that separates the pre and postsynaptic terminals (that is, the connecting parts of two neurons).
- Impulses can't 'jump' the synaptic cleft, instead, they send neurotransmitters across.
- The postsynaptic membrane: The dendrite of the next neuron, after the synapse. When neurotransmitters bind to receptors on the postsynaptic membrane, ion channels open, allowing positively charged ions to enter. If enough arrive, a new impulse is formed and the message is passed on.
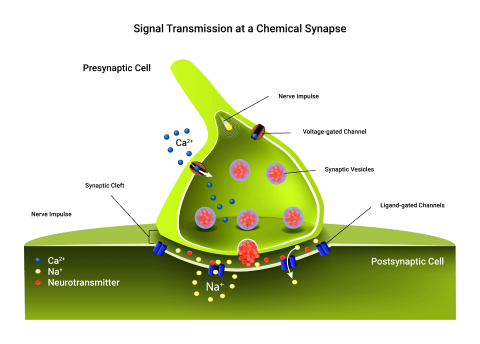
How do nerves fire?
Electrical impulses travel from motor neurons to muscle fibres. This is what makes movement possible. The steps in this process are:
- Electrical impulse (action potential) flows down the body of the presynaptic neuron.
- This positive charge (voltage) encourages ion channels on the membrane of the synaptic end bulb to open and let in calcium Ca2+.
- The influx of positive Ca2+ ions triggers the packages (vesicles) of neurotransmitters to move from the middle of the bulb to the outside and spill their contents into the synaptic gap.
- The neurotransmitters then bind to receptors on the postsynaptic terminal (bulb) and trigger the receptors to open and let in the Na+ ions which then, in turn, continue the positive charge from one neuron to the next. And so the cycle continues!
Watch
The following video shows a concise rundown of the communication process at the synapse. It is a little longer than other videos and touches on aspects in more detail that you are expected to learn. However, it is a great overview that will assist in cementing the concepts you have learnt so far about nerve anatomy and its structure and function.
All or none principle
Action potentials are electrical impulses. Where does the electricity come from?
Ions in the body are electrically charged. Sodium Na+ and Potassium K+ both have one positive charge. Calcium Ca2+ has 2 positive charges. And Chloride Cl- has one negative charge.
When a neuron is at rest, it is not sending an electrical signal and only some ions, like Potassium K+, can easily cross the cell membrane. The average resting membrane potential (charge) of a neuron is -70mv. To become electrically stimulated (excited), it needs to become more positive (or less negative). This happens via a process known as depolarisation.
Depolarisation occurs when a nerve increases its positive charge. This occurs as positively charged ions (e.g., sodium Na+/ calcium Ca2+) enter the nerve cell. A nerve must depolarise if it is to fire. The depolarisation must be enough to reach the neuron's threshold, so it must raise the neuron's resting membrane potential above -55mV
When the neuron's positive charge returns towards its resting potential, positive ions flow out of the neuron and as a result, its charge becomes more negative (less positive), returning towards -70mv. This is repolarisation.
The all-or-none law is the principle that the strength of the response of a nerve cell or muscle fibre is not dependent on the strength of the stimulus. If the stimulus is above a certain threshold, a nerve or muscle fibre will fire. For any given neuron, there are no big or small action potentials - they are all the same size.
The basics of the all-or-none principle are that the action potential must reach a certain threshold (-55mV) in order to fire. If it does not reach this, the neuron will not fire. It is all or nothing.
Watch
This video gives a thorough rundown of the before, during, and after action potential generation. Again, it is a little long and has more detail than required at this point. However, sometimes it pays to dig a little deeper to understand the surface knowledge.
Motor Divisions
The motor divisions of the nervous system include:
- Somatic division
- Autonomic division
- Parasympathetic division
- Sympathetic division
Study the following diagram to see how the motor divisions align with the central and peripheral nervous system divisions.
Motor division
Once a decision has been made by interneurons, motor neurons take and transmit the impulses to the body's effectors, such as the muscles, organs and glands.
The motor division of the PNS (peripheral nervous system) is divided into:
- Somatic nervous system (voluntary)
- Automatic nervous system (involuntary)
Somatic division
The somatic division includes nerve pathways of the PNS that lead to skeletal muscle. It creates voluntary movement.
Autonomic nervous system
The autonomic nervous system controls the body's involuntary internal functions:
- Heart rate
- Blood pressure
- Blood distribution
- Respiration
- Digestion
- Production of saliva, sweat and tears
- Urination
The automatic division can be split into two further divisions:
- Sympathetic division
- Parasympathetic division
Sympathetic division
The sympathetic division prepares the body for expending energy. It is used in emergencies or stressful situations and is also known as the 'Fight, Flight, Fright' response. E.g, flinching when someone shouts, when a ball flies towards you, breathing fast when nervous, and sweating in a job interview.
Parasympathetic division
The parasympathetic division is the body’s housekeeping system. It has a major role in digestion, producing urine, glandular secretion, and conserving energy. It is most active under ordinary restful conditions and is also known as the 'Rest and Digest' system. E.g., digesting food, sleep and wake cycles, and regulating breathing and heart rate.
Sensory Receptors Involved in Sport
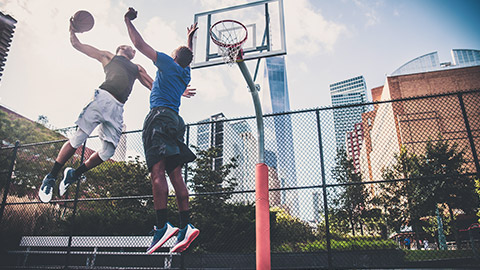
Sensory receptors play vital roles in sport and exercise. They enable us to know where our body parts are in space, help our body determine oxygen requirements during aerobic exercise, and keep us safe by helping to avoid soft tissue injuries. Let's focus on four key sensory receptors:
- Chemoreceptors
- Joint kinaesthetic receptors (JKR)
- Muscle spindle
- Golgi tendon organ
Chemoreceptors
These receptors monitor and respond to changes in the chemical environment within the muscle (e.g., Oxygen O2 and Carbon Dioxide CO2 levels, and acidity pH).
They provide the central nervous system (CNS) with information on the metabolic rate of muscular activity. An example of this is reporting increasing oxygen requirements for muscles when exercise begins. The chemoreceptors alert the CNS to change breathing and heart rate to match requirements.
Joint kinaesthetic receptors (JKR)
Located in joint capsules, joint kinaesthetic receptors (JKRs) are sensitive to joint angles and rates of change (of joint angle) so they can sense the position and movements of the joints. These receptors give feedback to the brain about where body parts are during movements. This 'feel' allows you to focus on other things (like kicking a ball or looking at your feet while you run) so you don't have to look at your body parts to know where they are.
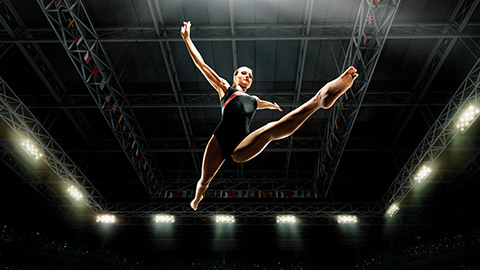
Balance is another great example of joint kinaesthetic receptors at work. The tiny adjustments made to keep balance show the reflex nature of these receptors. E.g., as we overbalance to one side, the JKRs send impulses that cause a reflex response resulting in muscle contraction to correct the overbalancing.
Muscle spindles (MS)
Muscle spindles (MS) are found alongside muscle fibres. They monitor both static and dynamic relative muscle length. They sense how much a muscle is stretched. If overstretched they cause the same muscle to contract. This set-up works to protect the muscle from overextension and tearing.
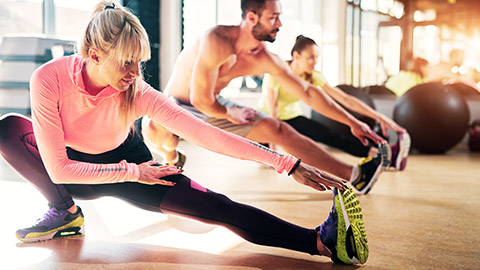
Muscle spindle (MS) and stretching
Stretching is a great example of muscle spindles in play. When stretching the hamstring muscle and the stretch is pushed too far, the MS will contract the hamstrings to protect them. This results in the knee bending.
Holding the stretch for 15-30 seconds can help a muscle spindle habituate (change its limits) so that repeating the stretch should result in a deeper stretch.
Patella tendon reflex test
Another example of the muscle spindles working to protect us is evident when performing the patella tendon reflex test.
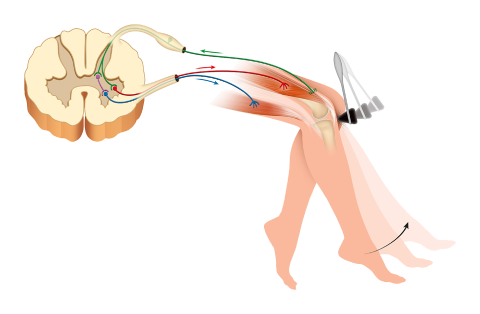
- The mallet strikes the patella tendon.
- This stimulus excites the nerve endings of the muscle spindle in the rectus femoris (quadriceps muscle).
- The muscle spindle fires impulses up the sensory (afferent) neuron to the spinal cord.
- A reflex response is fired immediately down the motor (efferent) neuron to the same muscle causing a contraction of the rectus femoris resulting in a fast extension of the knee.
- At the same time, the antagonist muscles (hamstrings- knee flexors) are relaxed.
The Golgi-tendon organ (GTO)
The Golgi-tendon organ (GTO) receptors are found at the muscle-tendon junction. They monitor the tension applied to a tendon. If they detect too much tension, they will inhibit (switch off) the contracting muscle to protect the tendon and stop it from tearing.
An example of the GTO in action is when someone fails in the gym. When attempting a heavy bench press, the pectoralis major may be overworked placing too much tension through the pec tendon. The GTO detects this and will switch off the pec major, causing failure.
The GTO has a lower threshold in new lifters. As you apply the progressive overload principle in your training, your muscles and tendons become stronger and the GTO will allow more stress on the tendon before acting.
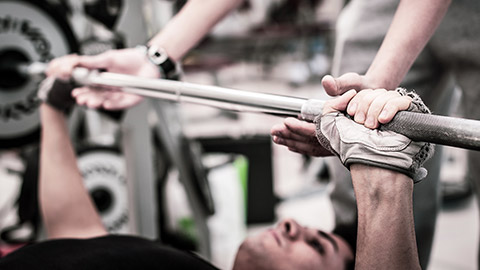
Nervous system adaptations to exercise
As we train, our nervous system adapts to the stimulus and develops its ability to communicate and perform movement. Have you ever noticed impressive strength gains in the gym when you start practising a new exercise? This may not be purely down to the strength of your muscles, but more likely improvements in your nervous system. In this section we will discuss what these adaptations are in more detail.
Nerve-muscle connections
The body’s ability to recruit motor units over time improves with training. When more motor units are recruited, it means that more muscles can contract simultaneously to produce more force – which in turn enables you to lift more weight.
Not only do the prime movers (you will learn more about this term in the muscular system topic) have more muscles involved, but the synergist muscles (assisting muscles) are also better activated over time, so again more force is able to be produced.
Efficiency of neural pathways increases as well – your brain is better able to communicate with the target muscles.
Additionally, our ability to summate (i.e send impulses to the correct muscles at the same time) also improves with practice.
Co-ordination
Have you noticed that the first time you perform a new movement, for example the barbell bench press, you were a little bit uncoordinated? Perhaps the barbell wobbled as you lowered it to your chest, and you struggled to keep it stable, or maybe you found you could not control the weight down smoothly? This is because your nervous system was new to the movement too, and your coordination needed work. Over-time, our nervous system learns to signal the correct target muscles in the correct sequence to allow for a more coordinated movement pattern. In the case of the bench press, you learn to use other body parts to keep you stable, for example, trying to keep your lower body still and your core braced, in order to focus on the movement in the shoulders and elbow joints and the contraction of the pectorals, deltoids, and triceps brachii.
Neuromuscular inhibition
When we perform movements such as lifting weights, our body has protective reflexes that may reduce the amount of force or tension able to be produced. Some of these we have covered so far are the actions performed by the Golgi tendon organ and the muscle spindles. What happens over a period of resistance training, is that these reflexes are dampened due to the body’s increased strength and familiarity with the movement. The more we practice and improve our resistance training techniques, the more we inhibit this neuromuscular inhibition, resulting in the ability to produce more force and lift more weights.
See this short video for a summary of these topics. Please note the video talks about muscle contractions as well which will be covered in the muscular system subtopic:
Check you have a good grasp of the nervous system before attempting your assessment and moving to the next topic.
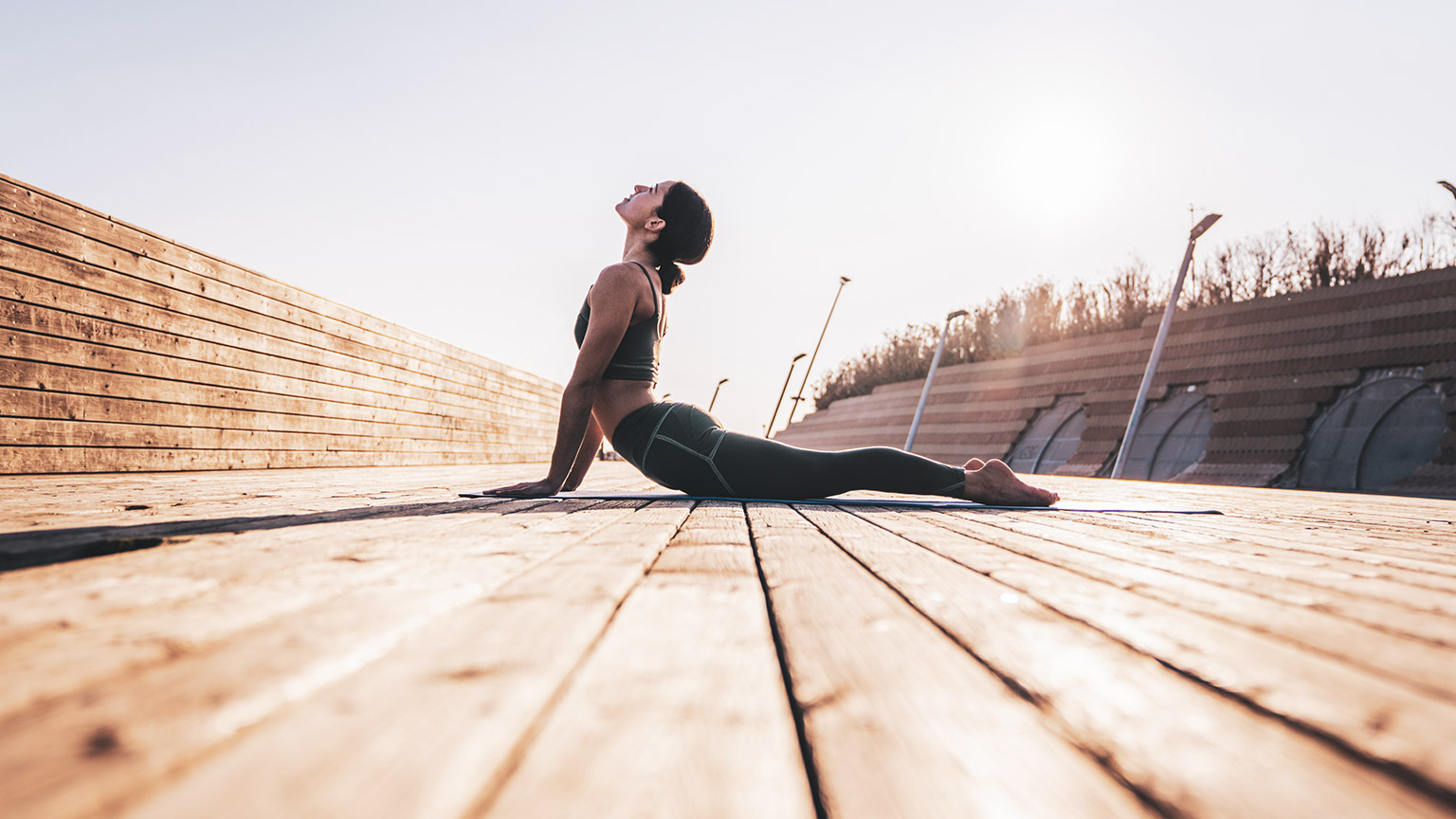