There are around 650 muscles in the body, making up about 40% of the body's weight. They allow us to move, breathe, digest, speak, and push blood around our bodies.
Bones provide our body with a framework, but without the muscular system and the nervous system, our bodies would be unable to move or carry out essential tasks. The muscular system (comprising of muscles and tendons) is constantly working, even when you are resting. Postural muscles keep you upright whilst sitting and standing, respiratory muscles keep you breathing, and the heart continually pumps blood around the body.
As well as aiding our whole-body movement, muscles help move food and food waste through our digestive system and produce most of the body heat we need to stay alive.
Well-conditioned muscles are necessary to live a healthy life. As a personal trainer, you will help your clients to work their skeletal muscles so they can run longer distances, lift heavier weights, or improve the strength and conditioning of specific muscles. Understanding the muscular system will help you instruct exercises, assess movement, correct technique, perform fitness testing, and design fitness programmes.
In this topic, you will learn about:
- Muscle types
- Muscle names and locations
- Functions of the muscular system
- Muscle attachment and interactions
- Muscle shapes
- Muscle structure
- Types of contraction
- Contraction and sliding filament theory
- Muscle fibre types
- Muscle fibre recruitment
- Muscles and targeted exercises
- Responses to training
Watch
Ashley completed the PT Level 4 course in 2022. In this video, she talks about what she took from the course, as well as shares some tips for studying anatomy and physiology.
Familiarise yourself with the major muscles. Using the correct and specific name for muscles will help you throughout this topic, your studies, and your future career.
Use the following images and the table to help you identify where these muscles are in your body. Practice naming these muscles until you can confidently identify all their names, locations, and actions.
These are the top 20 muscles you will refer to in your fitness career.
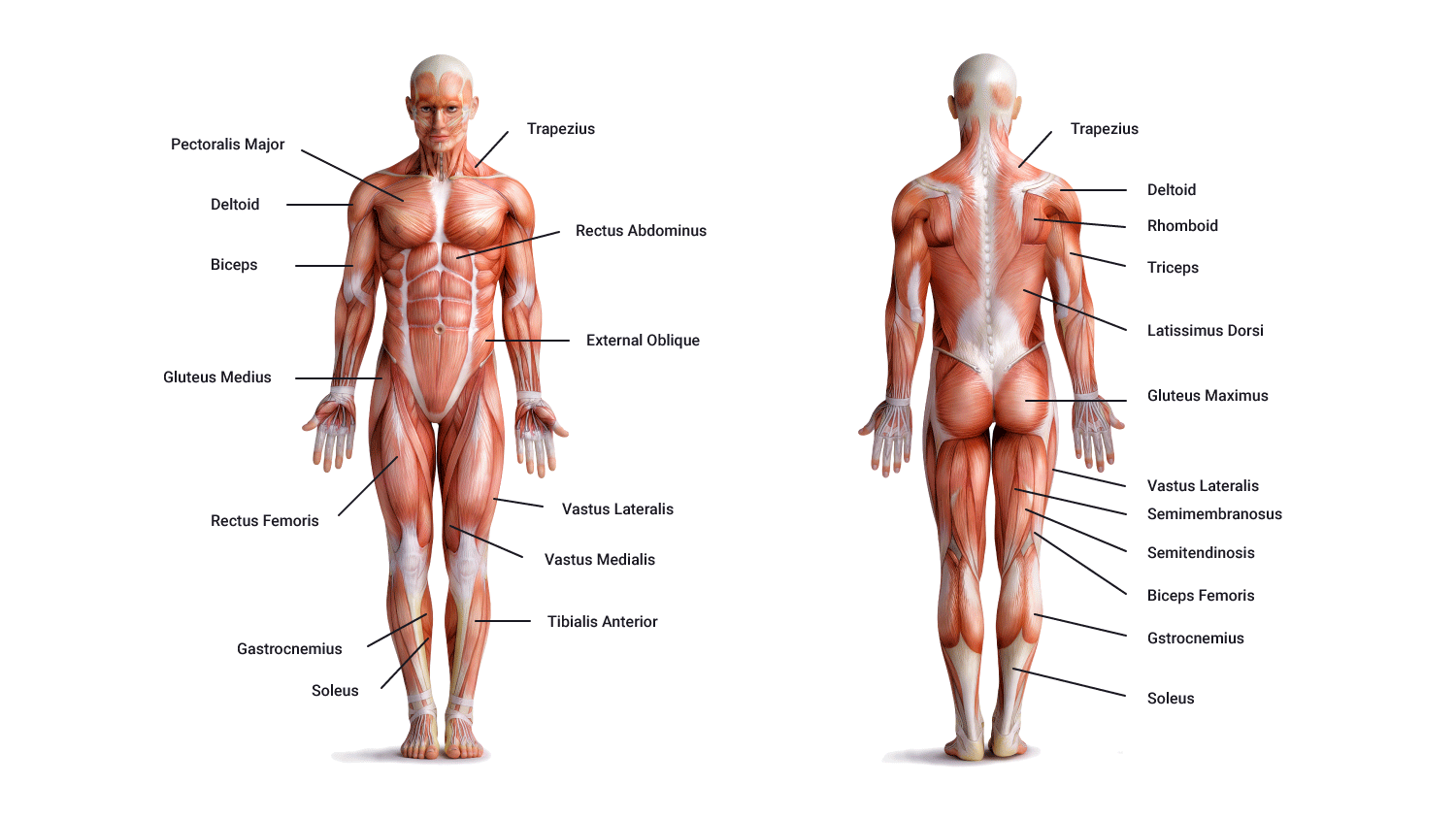
Muscle group | Muscle | Major action of muscle |
---|---|---|
Calves | Gastrocnemius | Ankle plantar-flexes, ankle flexes knee |
Soleus | Plantar-flexes ankle | |
Hamstrings | Biceps femoris | Flexion |
Semitendinosus | Flexion | |
Semimembranosus | Extension | |
Gluteals | Maximus | Extension |
Medius | Abduction | |
Chest | Pectoralis Major | Horizontal adduction |
Back | Lattisimus dorsi | Adduction Extension |
Arm | Biceps Brachii | Flexion |
Triceps Brachii | Extension | |
Quads | Rectus Femoris | Flexion |
Vastus lateralis | Extension | |
Vastus medialis | Extension | |
Abdominals | Rectus abdominis External obliques |
Flexion extension rotation Stabilisation of spine |
Shoulder | Deltoids | Flexion abduction extension |
Trapezius group | Elevation retraction depression | |
Tibialis anterior | Ankle dorsiflexion Inversion of foot |
|
Hip adductors | Adduction Medial rotation Flexion of thigh |
Try it out
Have a play with Poke-a-muscle. This is a game from Anatomy Arcade which helps you learn your muscles while playing a game.
Watch
It's a bit cheesy, but if you learn through music, the muscle song might be for you...
Muscle types
There are 3 main classifications of muscle in the human body:
- Skeletal
- Smooth
- Cardiac.
These 3 types are illustrated in the following diagram. You'll notice they are structurally different.
Skeletal muscle
These muscles are attached to the bones in your body - hence the name skeletal muscle. These muscles help you move around and manipulate your environment (e.g., to pick things up, drive a vehicle, or play sports). We have control over these muscles, so they are classified as voluntary. We voluntarily move them. We consciously control skeletal muscles via the somatic nervous system.
Skeletal muscles are also referred to as striated muscles because they appear striped under a microscope. This is due to myofilament alignment.
Interestingly, skeletal muscles are 40-50% of total body mass for males, but 30-40% for females.
Smooth muscle
Your smooth muscles are the muscles that protect special organs in your body, like your stomach, intestines, and lungs. They don't move when we want them to, instead, they are involuntary. You cannot control smooth muscle consciously (directly). Instead, it is regulated by the autonomic (involuntary) division of the nervous system.
Smooth muscle appears smooth under a microscope. It is not striated.
Most smooth muscle is found around and within internal organs and walls of hollow structures. E.g., in the walls of the digestive, respiratory and urinary systems and in the walls of veins and arteries.
The contraction of smooth muscle is 'wave-like' and called peristalsis.
Watch
The following video explains how smooth muscle contracts via peristalsis.
Cardiac muscle
The 3rd type of muscle is cardiac muscle. Cardiac muscles are a special type of muscle, found only in the heart. They must work automatically and constantly. Cardiac muscle never needs rest. It is classified as involuntary.
The cardiac muscle is responsible for moving blood through the heart's chambers and pumping it to all parts of the body.
Cardiac muscle, like skeletal muscle, is also striated.
Skeletal muscle shapes
Muscles come in many varied shapes and sizes depending on their location and function, as you can see from the following diagram.
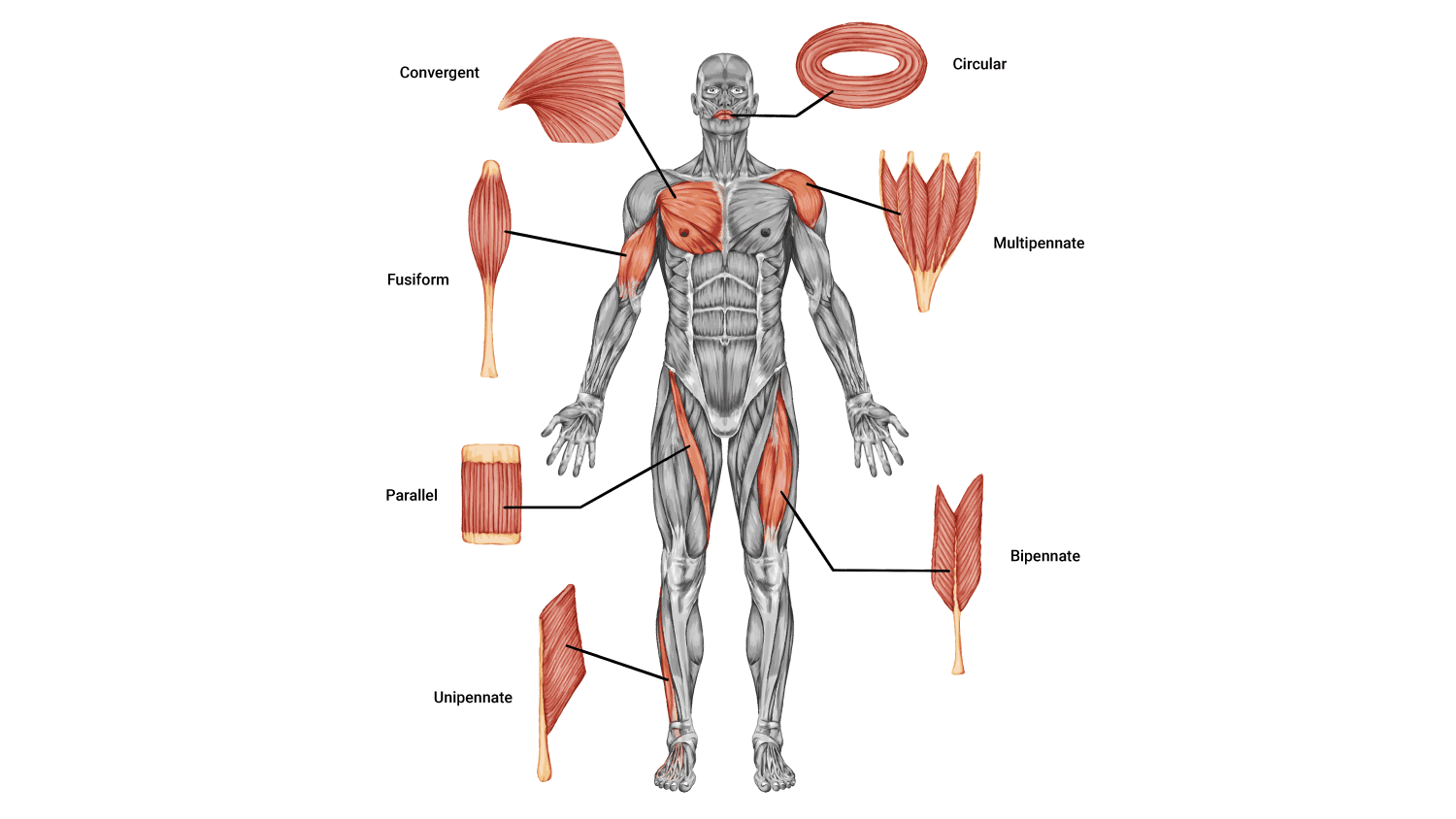
Fusiform | Fusiform is when all fibres run in one direction and taper at each end. For example, biceps brachii, quads, and hamstrings. |
---|---|
Parallel | Parallel is when the fibres run in one direction and can be separated into compartments. For example, the rectus abdominous. |
Convergent | Convergent is when the fibres have a wide origin tapering off to a narrow insertion. For example, the pectoralis major and adductor muscles. Allows contraction from different angles. |
Unipennate | Unipennate is when the fibres run in one direction but are of different lengths and graft directly onto the bone. For example, palmer interossei (adducts fingers). |
Bipennate | These feather-like fibres run in two directions and attach to a central line of fascia. For example, the rectus femoris (quadricep). |
Multipennate | Multipennate fibres run in multiple different directions allowing multiple movement planes. For example, the deltoid. |
Circular | These ring-shaped muscles squeeze shut or open and are often known as 'sphincters. For example, the obicularis oris (pinches mouth closed) and the obicularis oculi (squeezes eye shut). |
Muscle Structure
Let's take some time to familiarise ourselves with the physiology and the microscopic structures of a skeletal muscle fibre. To get a complete understanding of muscle structure we will start from the outside and work our way in.
Tendons
Tendons are tough cords of collagen fibres that grow directly from the muscle fibres which attach muscle to bone. Without this link, there would be no limb movement.
Fascia
The fascia is a protective connective tissue surrounding muscles, bones, organs, nerves, blood vessels, and other structures. It also separates the various tissues from one another.
The facia holds muscles with similar functions together. It allows the 'free' movement of muscles. I.e., it carries structures such as nerves, blood vessels, and lymphatic vessels and fills the spaces between muscles.
It is also responsible for maintaining structural integrity, providing support and protection, and acting as a shock absorber.
3 layers of connective tissue extend from the facia, and are named based on their location:
- Epimysium (Epi~ on/upon/before): This is the outer layer of connective tissue surrounding the whole muscle.
- Perimysium (Peri~ around/enclosing): This internal layer surrounds groups of 10-100 muscle fibres, bundling these fibres into clusters or bundles called fascicles.
- Endomysium (Endo~ within). This layer surrounds each individual muscle fibre and separates each one from another.
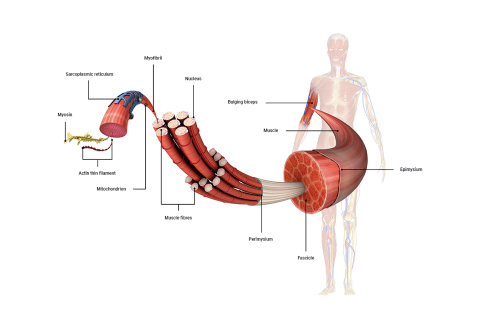
Muscle fibres
Muscles are made up of a large number of individual structures called fibres. The number of fibres present in a muscle varies depending on the size and function of the muscle. Muscle fibres range in diameter from 10 to 80 micrometres which are practically invisible to the naked eye. However, they can be more than 35 cm long.
Fascicles
Muscle fibres are known as myocytes (Myo~=muscle). Within a muscle, they are organised into bundles known as fascicles. Each fascicle is likely to be made up of one type of muscle fibre (for example, slow twitch and fast twitch).
Sarcolemma
A muscle fibre surrounded by a plasma membrane is called the sarcolemma. Inside the sarcolemma, there are myofibrils which are rod-like structures running the length of the muscle fibre.
There is also a gelatine-like substance that fills the spaces between the myofibrils. This is the sarcoplasm. This is where fuels are stored, such as glycogen, amino acids, and triglycerides. You can see these in the following diagram.
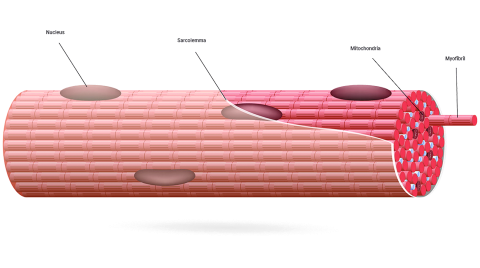
Sarcoplasmic reticulum
The sarcoplasmic reticulum is a longitudinal network of tubules (green in the following picture). These lie parallel to the myofibrils and loop around them.
It is a storage site for calcium (Ca2+), essential for muscle contraction.
Myofibrils
Each muscle fibre consists of several hundred to several thousand myofibrils. Myofibrils are the contractile elements of skeletal muscle. They appear as long strands of even smaller sub-units called sarcomeres.
Sarcomere
Myofibrils are broken up along their lengths into sarcomeres. It is the interaction between the 2 myofilaments found within these repeating sub-units that we need to understand to fully appreciate how a muscle contracts.
Myofilaments
Myofibrils are composed of 2 types of myofilament called myosin (also known as contractile proteins) and actin:
- Myosin is a thick protein filament.
- Actin is a thin protein filament.
These myofilaments interact to cause the contraction of a sarcomere, which translates to the contraction of a myofibril, muscle fibre and ultimately the muscle itself. Let's look further at these.
Myosin
Each myosin filament contains several hundred globular heads, which protrude from the myosin filament to form cross-bridges that interact during muscle contraction with special active sites on the actin filaments. These globular myosin heads are shown in purple on the following diagram.
Actin
Each actin filament contains active sites to which the myosin head can bind. At rest, these active binding sites are covered by a barrier known as tropomyosin. Tropomyosin is guarded by a molecule known as troponin.
Muscle Attachment and Interactions
Skeletal muscles can attach to bones in 2 different ways.
- Direct attachment
- Indirect attachment
Let's look at what these terms mean.
Direct attachment
Direct attachment is where the muscle grafts directly into the bone. This occurs in places where tapering into a tendon is not possible, such as in the muscles of the jaw and forehead. It usually occurs in muscles that need to attach along the length of a bone.
Indirect attachment
Indirect attachment is where skeletal muscles attach to the bone via tendons (as is the case of most muscles). Tendons are tough cords of collagen fibres that grow directly from the muscle fibres.
Muscle attachment sites
Study the terms and their locations in the following diagram.
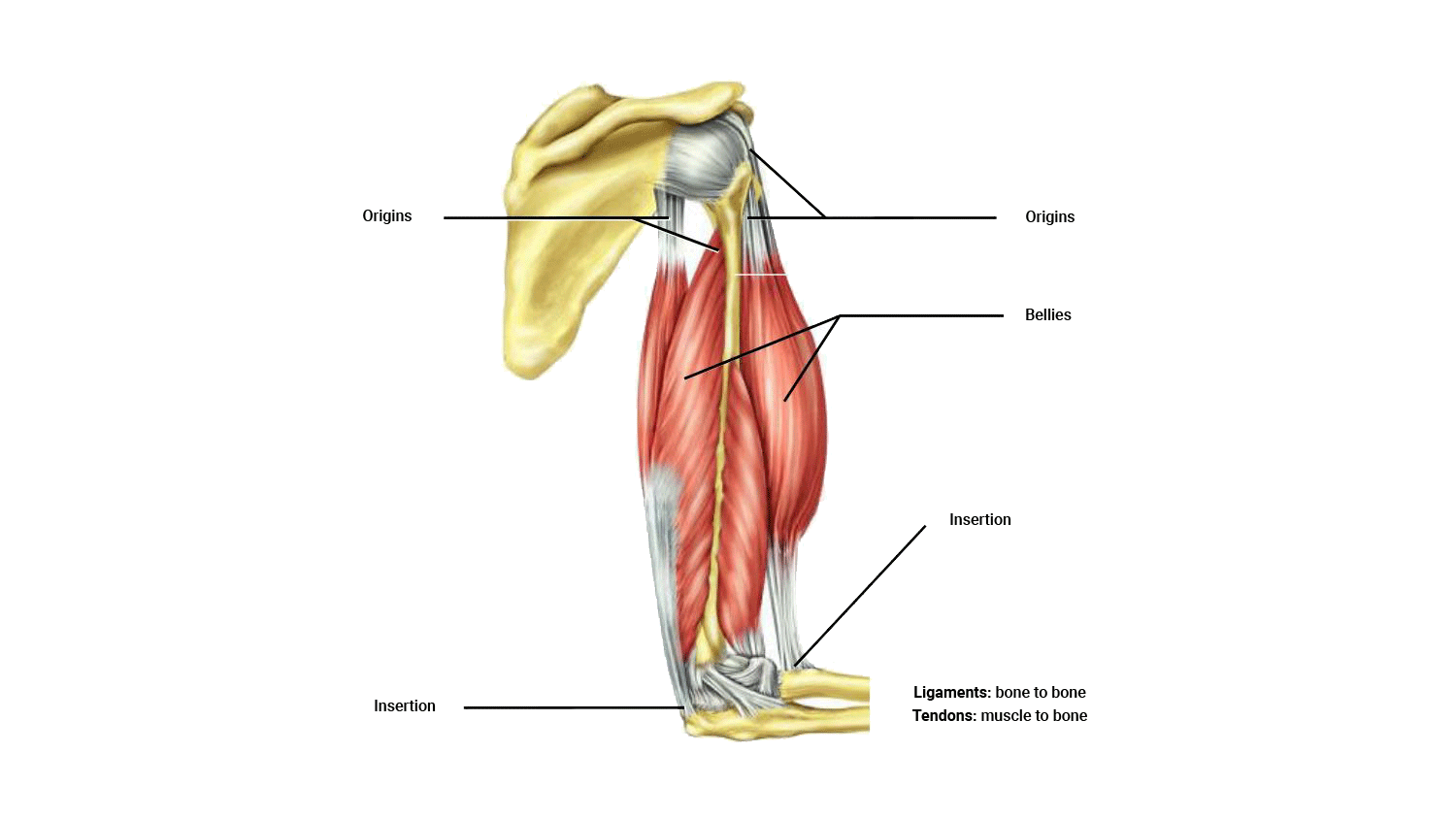
Origin | The anchor for contraction does not move during contraction. It is immovable and closer to the midline (proximal). The term origin refers to the site of attachment of the muscle’s tendon to the stationary bone. |
---|---|
Insertion | This refers to the attachment site of the muscle’s tendon to the movable bone. It is more distal. |
Belly | Also known as 'body'. This refers to the fleshy part (region) of the muscle between the muscle tendons. This is typically the widest part of the muscle. |
When the muscle contracts, it brings the insertion closer to the origin (think of a bicep curl involving the bicep). When the action is performed, the distal insertion on the forearm is pulled towards the proximal origin in the shoulder.
Muscle interactions
Muscles can, but seldom do work in insolation. They work as a group. The muscle contracting is dependent on other muscles to help with contraction. The following is a list of partnership styles muscles have together. It is very important to be comfortable with these and keep these relationships in mind when prescribing exercises.
- Agonist
- Antagonist
- Fixator
- Synergist
Agonist and antagonist
These terms are important in understanding how muscles on opposite sides of a joint interact together.
- Agonist: The prime mover, the targeted muscle or main muscle working in a given exercise. It is responsible for the movement and will contract to produce the desired movement.
- Antagonist: The muscle that opposes the agonist (provides resistance and stabilises movement). It needs to relax to allow the agonist to contract.
The antagonist's role is often confused. It generally only plays a role when moving without resistance. When lifting a weight, the weight itself is the antagonist (providing resistance against which the agonist works).
When no external resistance is present. For example, when using your bicep to lift a cup of coffee, the triceps must provide some resistance so that the biceps have something to work against. Without this antagonistic resistance, it would be difficult to control the movement.
Synergists and fixators
While the agonist and antagonist are of primary importance in movement, they can't do it on their own. Other muscles play vital support roles that enhance movement quality.
- Fixator: The fixator muscles stabilise the bone/joint during contraction. It fixes the joint at the origin of the muscle. These muscles need to be stable while performing a movement. It keeps the movement in one plane and ensures movement is efficient.
- Synergist: The synergist muscle assists the prime mover by reducing unnecessary movements. It supports the movement of the agonist.
Example of muscle interaction
Can you identify what muscles act as the agonist, antagonist, fixator, and synergist during a bicep curl?
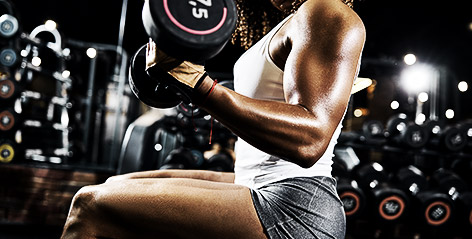
- Agonist = bicep brachii
- Antagonist = tricep brachii
- Fixator = rotator cuff muscles act as fixators, stabilising the shoulder joint
- Synergist = brachialis supports the movement as it crosses the elbow joint as well
Muscles are made up of three main fibre types.
- Slow-twitch (type 1)
- Fast-twitch A (type 2a) (intermediate)
- Fast-twitch B (type 2b)
A person's muscle fibre makeup is predominantly determined by genetics.
Training does not impact the types of fibre a person has.
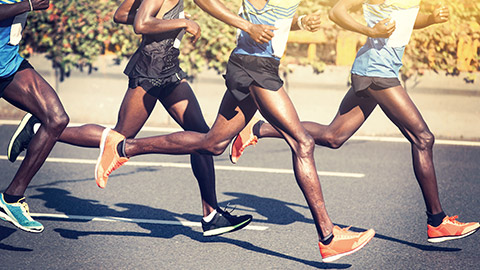
Let's look at the different muscle fibre types.
Slow-twitch (type 1)
Slow (oxidative) fibres are generally used for posture and low-intensity movement, like long-distance or slow activities. Sports and exercises which use slow-twitch muscle fibres include marathons, distance running, swimming, cycling, power walking, and endurance training. The soleus and postural muscles of the back are slow-twitch.
Intermediate (type 2a, fast-twitch)
Fast oxidative-glycolytic fibres (FOG) contain characteristics of both type 1 and type 2b fibres. Rather than used for specific tasks, these fibres can be trained to take on more of the characteristics of one of the other fibre types.
Fast-twitch (type 2b)
Fast glycolytic fibres (FG) are for specialised use in 'quick energy' or power-based activities and exercises. Sports and exercises which use fast-twitch (type 2b) include powerlifting, sprinting, jumping, stretch and agility training.
Summary of characteristics
The difference in muscle fibre characteristics can affect how a muscle presents in terms of colour. Slow-twitch fibres are red, intermediate (fast-twitch type 2a) are red-pink, and fast-twitch (type 2b) are white/pale.
The characteristics of the 3 muscle fibre types are shown in the following table.
Slow Oxidative Fibres (SO) - Type 1 | Fast Oxidative-Glycolytic Fibres (FOG)- Type 2a | Fast Glycolytic Fibres (FG)-Type 2b | |
---|---|---|---|
Structural characteristics | |||
Myoglobin content | Large amount | Large amount | Small amount |
Mitochondria | Many | Many | Few |
Capillaries | Many | Many | Few |
Colour | Red | Red-pink | While (pale) |
Motor unit size | Small | Medium | Very large |
Functional characteristics | |||
Capacity for ATP generation | High | Intermediate | Low |
Contraction speed | Slow | Fast | Very Fast |
Fatigue resistance | High | Intermediate | Low |
Order of recruitment | First | Second | Third |
Location where fibres are found | Postural muscles of the back and neck | Lower limb muscles | Upper limb muscles |
Primary function of muscles | Maintaining posture & aerobic (with O2) endurance activities | Walking, sprinting | Rapid, intense movements of a short duration |
Maximum duration of use | Hours | <30 mins | <1 minute |
Activity used for | Aerobic | Long-term Aerobic | Short-term aerobic |
Key anatomical terms
- Mitochondria: Mitochondria are found within the muscle cell and are referred to as the 'powerhouse' of a cell. The function of mitochondria is to produce ATP for muscle energy using the aerobic system.
- Myoglobin: Myoglobin is a red pigment that is a storage site for oxygen within the muscle. Skeletal muscle fibres have a high myoglobin content and are referred to as 'red muscle fibres'. Muscles with high myoglobin appear darker.
Watch
The following video explains slow- vs fast-twitch muscle fibres in a really simple way, looking at the science of dark and white meat in poultry.
Humans also have darker and lighter meat. Some of our muscles, like the soleus in the lower leg are almost all slow-twitch fibres. Others like those controlling eye movements are made up of only fast-twitch fibres. Muscles adapt to the function they are required for. The majority of human muscles contain a mixture of both slow and fast fibre types.
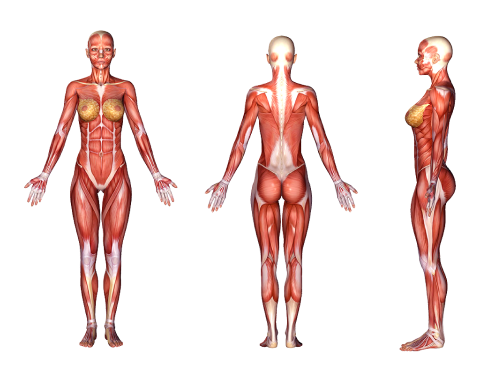
Properties of muscle
Unique properties enable muscle tissue to function and contribute towards achieving and maintaining homeostasis.
- Contractility: The ability of the muscle to shorten with force. When a skeletal muscle contracts, it generates tension, causing movement of the structures it is attached to (usually bone). It is important to note that muscles only pull on bones, they do not push.
- Excitability (electrical excitability): The capacity of skeletal muscle to respond to stimulus. Muscles are stimulated by nerve impulses (electricity) sent by the nervous system and chemicals such as neurotransmitters and hormones distributed by the blood.
- Extensibility: A muscle's ability to be stretched within its limits without being damaged.
- Elasticity: The ability of the skeletal muscle to 'recoil' to its original resting length after contraction or extension.
- Adaptability: Muscle will enlarge in response to increased work, or waste away if it is deprived of work.
Functions of muscle
Due to their ability to contract and relax, muscular tissues have the following functions.
- Movement: This includes whole-body movements like walking or running and more precise movements such as picking up a pencil and nodding your head. It also helps with things such as respiration, urination, and digestion.
- Posture (support/stability): Muscles support internal organs, stabilise the spinal column and maintain posture by holding joints in place.
- Communication: Muscles allow facial expressions, body language, writing, and speech.
- Control of body passages: Achieved by circular muscles called 'sphincters'. For example, to prevent outflow from organs and enable the temporary storage of substances in organs such as the urinary bladder and stomach.
- Protection: Protect major internal organs by absorbing shock and reducing friction in joints. Sphincters of the eyes, mouth and other orifices also prevent outside foreign bodies from entering the body (e.g., dust in your eyes).
- Heat production: Approximately 85% of body heat is produced by muscles. As muscles contract, heat is produced through a process called thermogenesis. Contrary to popular belief, this heat production is not the result of friction. When muscle contracts and uses energy, heat is produced as a by-product of cellular metabolism. When you are cold, your body will initiate this without conscious thought (shivering).
Contraction and Sliding Filament Theory
For muscles to perform their functions, they typically need to contract which requires innervation by the nervous system which carries impulses to the muscles. The process of muscle contraction requires a few different elements/components and steps.
Motor neurons carry impulses to muscles. In this section, we will look at the connection between motor neurons and muscle fibres. Muscle contraction involves the nervous system. You may find it helpful to review the subtopic on nerve impulses.
Note that this section is quite complex and it is unlikely you will have to explain this to a client. However, the knowing how and why muscles contract is foundational and absolutely crucial. It helps us to understand the demand and stress we place on the muscles during exercise.
A motor unit
Muscle fibres never fire in isolation. Instead, a group of fibres is innervated by a single motor neuron.
A motor unit is defined as one motor neuron and all the muscle fibres it innervates.
Parts of a motor unit:
- One motor neuron
- Muscle fibres
- The neuromuscular junctions.
Structure of a synapse
A synapse is the site of communication between a neurone and another neurone or a neurone and an effector cell, allowing communication.
These meeting points allow impulses to be passed between the different neurons. In the case of the motor neuron meeting a muscle, the post-synaptic membrane is the muscle fibre. Instead of being known as a synapse, this meeting point is known as a neuromuscular junction.
Neuromuscular junction
As you can see in the diagram, the synaptic cleft (gap) still exists in this meeting of nerve and muscle. As impulses (action potentials) cannot jump the gap, we require a neurotransmitter to cross the cleft to stimulate a charge on the other side. In this case, the neurotransmitter is known as acetylcholine (Ach).
Motor neurons arriving from the spinal cord arrive at the pre-synaptic terminal causing the release of the neurotransmitter acetylcholine (Ach) across the neuromuscular junction. Acetylcholine then binds to receptors on the muscle fibre surface.
A new electrical charge is then transmitted through the full length of the muscle fibres in that motor unit. These impulses could potentially lead to contraction.
We will now do an overview of a neurone to muscle communication (pre-contraction). Think of this as part 1 of muscle contraction with part 2 being the sliding filament theory.
Pre-contraction: How nerves fire
Electricity (stimulus) is the main currency required for muscle contraction to occur, along with sodium(Na+), Calcium (Ca2+), ATP ( Adeonsidetriphosphate- energy currency), and the neurotransmitter acetylcholine (Ach). The main steps of pre-contraction:
- Electrical impulse (action potential) flows down the body of the presynaptic neuron.
- This positive charge (voltage) encourages ion channels on the membrane of the synaptic end bulb to open and let in calcium Ca2+.
- Ca2+ triggers the packages (vesicles) of neurotransmitters to move from the middle of the bulb to the outer membrane and spill their contents into the synaptic gap. Note: Sodium Na+ is already 'floating' in the gap.
- The neurotransmitters then bind to receptors on the postsynaptic terminal (bulb) and trigger the receptors on the postsynaptic membrane to open.
- Sodium ions (Na+) floating around in the synaptic gap can now flow into the postsynaptic membrane and flood the membrane with a positive charge. Note: This positive charge needs to be of a sufficient level to 'depolarise' the membrane (that is, to stimulate the membrane).
Sliding filament theory
The process of muscle contraction is described by the 'Sliding filament theory'. The theory suggests that the two myofilaments actin and myosin slide past each other during muscle contraction. You can read more about the components involved in the sliding filament theory by expanding the accordions.
The process
Let's review and build on what we have learnt so far.
- The process begins with the arrival of the motor impulses at the neuromuscular junction.
- The neurotransmitter acetylcholine (Ach) is released across the synaptic cleft which binds to receptors (channels) on the postsynaptic membrane.
- These channels then open and permit the entry of sodium (Na+) into the postsynaptic membrane. The arrival of sodium (Na+) increases the positive charge within this region and as a result, stimulates an action potential.
- This action potential travels throughout the portions of the muscle and heads towards the sarcoplasmic reticulum (huge calcium storage found wrapped around the myofibrils within a muscle fibre) which becomes stimulated by these new impulses.
- The result of this is a release of calcium (Ca2+) into the myofibrils of the muscle. The contraction process continues via the involvement of the:
- contractile proteins actin and myosin
- regulatory proteins troponin and tropomyosin
- energy source, known as ATP.
- Calcium stimulates the troponin to trigger tropomyosin to move (tropomyosin covers the myosin-binding site when a muscle is not contracting) and by doing so, reveals the myosin-binding site on the actin.
- ATP then binds to the myosin heads which gives them the energy they need to move and bind to their site on actin allowing myosin heads to form cross-bridges with actin and start the contraction process.
- When myosin forms cross-bridges with actin, the two filaments slide past one another as actin is pulled towards the centre of the sarcomere. It is thought that the myosin heads (cross-bridges) pull the actin filaments towards the centre of the sarcomere, shortening the muscle. The tilting of the myosin head is called the power stroke.
The following diagram presents this information visually.
Muscle shortening
Immediately after the power stroke, the myosin head breaks away from the active site, rotates back into its original position and attaches to a new site further along the actin filament.
Repeated attachments, power strokes and re-attachments cause the filament to slide past one another and muscle shortening occurs. Around 3 to 5 power strokes are performed by each myosin head per second of contraction!
Muscle relaxation: Ending a muscle contraction
A muscle will continue to contract if there are sufficient levels of Ca2+, ATP, and electrical impulses (action potentials) arriving. After the last action potential arrives, with the support of ATP, calcium (Ca2+) is pumped back into the sarcoplasmic reticulum where it is stored in preparation for the arrival of the next action potential. This requires energy in the form of ATP. Note that ATP is needed for both muscle contraction and relaxation.
During the muscle relaxation phase, tropomyosin moves back into its 'resting position' covering the myosin-binding sites on actin, preventing cross-bridge forming. Until the next action potential, there are insufficient volumes of calcium (Ca2+) and ATP, therefore muscle contraction is unable to occur.
Steps required to aid muscle relaxation
- The enzyme acetylcholinesterase breaks down and decomposes acetylcholine. This neurotransmitter can no longer stimulate the muscle in a decomposed state.
- Any remaining or residual calcium ( Ca2+) is pumped back into the sarcoplasmic reticulum (with the aid of ATP).
- Troponin and tropomyosin return to their original positions, re-covering the myosin-binding sites on actin.
- ATP is broken down into its constituents which results in the disconnection of the cross-bridge connection between actin and myosin to break down (no longer connecting these two contractile proteins).
- Muscle fibre relaxes.
Watch
The following video provides a simple summary of muscle contraction with entertaining analogies to support our understanding of this process. You do not need to know this in-depth but it is important that you have a grasp of the process overall.
Types of Contraction
Muscles can contract in a number of different ways. There are 3 main contraction types:
- Isotonic contraction (concentric and eccentric phases)
- Isometric contraction
- Isokinetic contraction
Isotonic contractions
Isotonic contractions are when the tension is the same, but the length changes and produces force. Examples of isotonic contractions include stair climbing, push-ups and squats to name a few. It can be either:
- Concentric: The ends of the muscle come closer together. The muscle creates force while shortening in length.
- Eccentric: The ends of the muscle move further apart. The muscle creates force while increasing in length.
These are commonly seen in gym lifts.
- The concentric phase can be thought of as the work phase where the weight is lifted, the phase of the movement which is overcoming gravity. Whenever a weight goes up in the gym, that is the concentric phase. Note that sometimes you 'pull-down' in the gym, to get the weight to lift, such as when you perform a lat pull-down.
- The eccentric phase is the release phase where weight is lowered under control, resisting the load or the effects of gravity.
When looking at a bicep curl in terms of concentric and eccentric phases, the concentric phase of a bicep curl would be raising the dumbbell towards the shoulder, away from the ground, overcoming gravity to lift the weight upwards, away from the floor. The eccentric phase would be the safe lowering movement, bending at the elbow, and controlling the movement back towards the floor/resting or starting position of the movement.
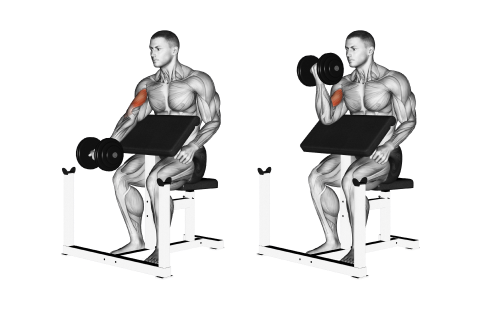
A common misconception...
Many people think that during a gym lift the agonist works on the 'lift' portion of the exercise, while the antagonist works on the down phase. For example, during a biceps curl the biceps work on the up phase and the triceps work on the down phase. This is incorrect!
Think about it for a minute. If the triceps worked on the down phase of a biceps curl the elbows would extend rapidly (instead of under control). We don't need to engage the triceps as gravity wants to return the weight towards the floor. Instead, the biceps must work (eccentrically) to control the rate of descent.
The same is true of any weighted gym lift, or movement against gravity. We use concentric contraction of the agonist to lift the weight, then eccentric contraction of the agonist to return the weight to the starting position under control. So the same muscles are targeted in both phases of the lift.
Isometric contraction
An isometric contraction is when the muscle produces force but does not change length, (e.g., a prone hold (plank) or wall sit). Tension is present. Muscles suited to endurance work would be best at this type of contraction. Postural muscles use isometric contractions to keep body parts upright (against gravity). E.g., back extensors and deep abdominals supporting the spine in sitting and standing.
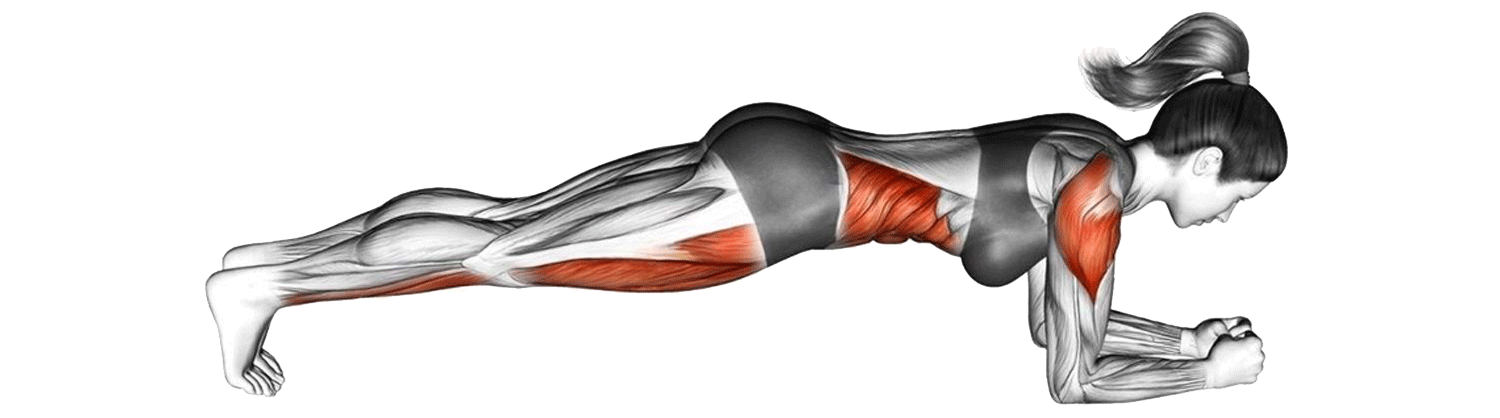
Isokinetic contraction
During this type of contraction, the length of the muscle changes but the force is produced at a constant velocity in both movement directions. Imagine doing a gym exercise but being resisted in both directions; e.g., if you are performing a seated leg extension followed by a seated hamstring curl on the same machine in one rep.
This type of training requires specialised machines. Typically, the amount of resistance is dictated by the force the athlete produces. I.e., the harder you push the more it resists keeping the velocity of the movement constant.
Isokinetic exercises are typically used in recovery and physical rehabilitation and these machines are rarely seen in a gym environment.
Checkpoint
Strengthen your learning by completing the practical activity.
Muscle Roles
Understanding the roles and contractions of skeletal muscles is vital to designing programmes that are specific to your client’s goals and abilities – as well as helps ensure good technique and safety.
Even during a basic movement like walking or squatting, many different muscles contribute to making the movement smooth and effective. Each muscle involved performs an appropriate type of contraction and has its own clearly defined role during the movement.
The 4 defined roles muscles take on during a movement are agonist, antagonist, synergist, and fixator.
Role | Definition |
---|---|
Agonist | Agonist muscles cause a movement to occur through their own contraction. They are the prime mover for an exercise. E.g., the agonist of the bench press is the pectoral group. |
Antagonist | Antagonist muscles are the opposite muscle group to the agonist. The antagonist typically relaxes, so it doesn’t prevent the agonist moving. At other times, it is actively working to slow down or stop a movement and may contract. E.g., the antagonist muscle group for the barbell curl is the triceps group. |
Synergist | Synergist muscles assist the prime mover in an exercise. E.g., the synergists for the bench press are the triceps and the anterior deltoid. Not all exercises will have synergist muscles assisting the agonist. If an exercise is an isolation exercise, then it only targets one muscle group. |
Fixator | Fixator muscles stabilise the body during an exercise to help the agonist function most effectively. E.g., the erector spinae are the fixators for the T-bar row. |
Let's look at the roles of the muscles involved in a barbell bench press.
Agonists and antagonists
During a barbell bench press, the pectoralis major (or "pecs") are the agonists. The pecs are responsible for the horizontal adduction of the shoulder joint and the extension of the humerus (upper arm bone) towards the chest.
The latissimus dorsi or "lats" and the rhomboids are the antagonists. They are responsible for retracting the scapula (shoulder blade) and extending the humerus away from the chest.
Synergists and fixators
The synergist muscles are the triceps and anterior deltoids in the barbell bench press. The triceps are responsible for elbow extension, which helps to push the weight up during the bench press. The anterior deltoids are responsible for shoulder flexion, which helps to bring the arms up and forward during the bench press.
The fixator muscle are the core muscles. The core muscles help to stabilise the trunk and maintain proper form during the bench press.
Identify muscle roles
Muscles and Targeted Exercises
At the start of this topic, you learnt the top 20 muscles for personal trainers to know. Now, build on your knowledge by considering the joints they act on, their major action, and a sample of exercises targeting these muscles.
This is just a taster of linking anatomy and physiology with exercise prescription. We will learn more about exercise prescription in future topics but the sooner you get familiar with linking anatomy, physiology, and exercise the better.
Try it out
As you go through the 'muscle top 20', identify the muscles on your own body and complete the exercises.
Muscle top 20
Calves
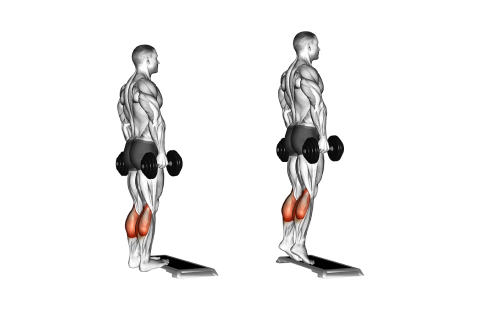
Joint/bone muscle acts on | Ankle/knee |
---|---|
Major action of muscle | Ankle plantar-flexes ankle Flexes knee |
Sporting/gym movement example | Standing calf raise |
Joint/bone muscle acts on | Ankle |
---|---|
Major action of muscle | Plantar-flexes ankle |
Sporting/gym movement example | Seated calf raise |
Hamstrings
Joint/bone muscle acts on | Knee |
---|---|
Major action of muscle | Flexion |
Sporting/gym movement example | Hamstring curls |
Joint/bone muscle acts on | Knee |
---|---|
Major action of muscle | Flexion |
Sporting/gym movement example | Hamstring curls |
Joint/bone muscle acts on | Hip |
---|---|
Major action of muscle | Extension |
Sporting/gym movement example | Deadlift |
Gluteals
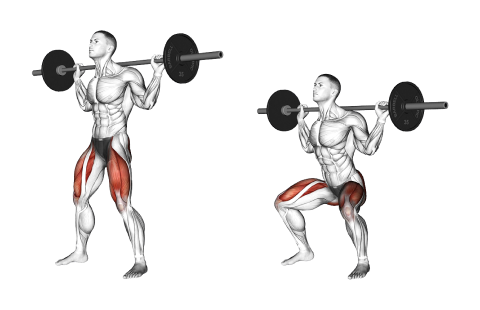
Joint/bone muscle acts on | Hip |
---|---|
Major action of muscle | Extension |
Sporting/gym movement example | Squats, leg press |
Joint/bone muscle acts on | Hip |
---|---|
Major action of muscle | Abduction |
Sporting/gym movement example | Hip abduction (cable) |
Chest
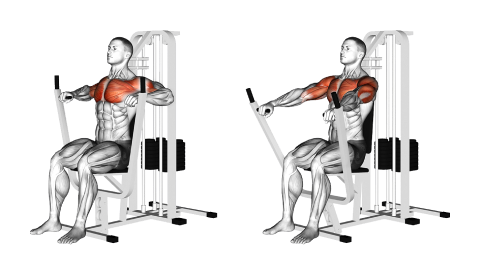
Joint/bone muscle acts on | Shoulder |
---|---|
Major action of muscle | Horizontal adduction |
Sporting/gym movement example | Chest press |
Back
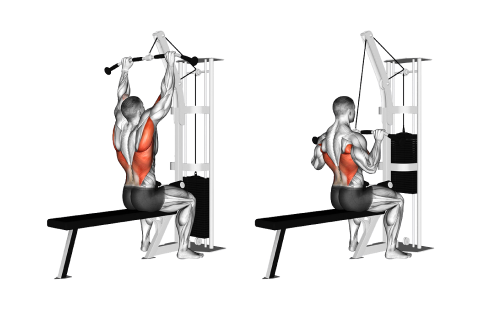
Joint/bone muscle acts on | Shoulder |
---|---|
Major action of muscle | Adduction Extension |
Sporting/gym movement example | Lat pulldown Seated row |
Arms
Joint/bone muscle acts on | Elbow |
---|---|
Major action of muscle | Flexion |
Sporting/gym movement example | Biceps curl |
Joint/bone muscle acts on | Elbow Shoulder |
---|---|
Major action of muscle | Extension |
Sporting/gym movement example | Tricep pulldown (cable) Straight arm pullback (cable) |
Quads
Joint/bone muscle acts on | Hip |
---|---|
Major action of muscle | Flexion |
Sporting/gym movement example | Hanging leg raise |
Joint/bone muscle acts on | Knee |
---|---|
Major action of muscle | Extension |
Sporting/gym movement example | Leg extension Squat |
Joint/bone muscle acts on | Knee |
---|---|
Major action of muscle | Extension |
Sporting/gym movement example | Leg extension Squat |
Abdominals
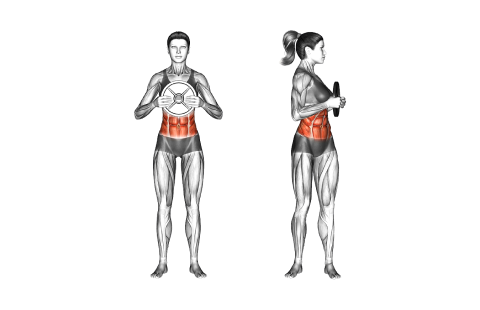
Joint/bone muscle acts on | Vertebra |
---|---|
Major action of muscle | Flexion Stabilisation of spine |
Sporting/gym movement example | Sit-ups Prone hold |
Joint/bone muscle acts on | Vertebra |
---|---|
Major action of muscle | Lateral flexion Rotation of trunk |
Sporting/gym movement example | Side bends Russian twist |
Shoulders
Joint/bone muscle acts on | Shoulder |
---|---|
Major action of muscle | Flexion Abduction Extension |
Sporting/gym movement example | Front raise Lateral raise Rear delt flies |
Joint/bone muscle acts on | Scapula |
---|---|
Major action of muscle | Elevation Retraction Depression |
Sporting/gym movement example | Shrugs Seated row |
Legs
Joint/bone muscle acts on | Ankle |
---|---|
Major action of muscle | Ankle dorsiflexion Inversion of foot |
Sporting/gym movement example | Walking, jogging, running Dorsiflexion with resistance band |
Joint/bone muscle acts on | Hip |
---|---|
Major action of muscle | Adduction Medial rotation Flexion of thigh |
Sporting/gym movement example | Seated hip adduction (machine) Standing hip adduction |
Muscle Fibre Recruitment
Individual muscles are a mixture of 3 types of muscle fibres (types 1, 2a, and 2b), but their proportions vary depending on the action of that muscle. Note that skeletal muscles, although a mixture, can only have one type of muscle fibre within a motor unit.
This section describes how muscle fibres are recruited.
Motor unit recruitment
Now that we understand how muscle fibre contracts, we need to broaden our focus as single muscle fibres never contract alone.
Muscles are made up of fascicles full of fibres. One motor unit can fire multiple fascicles of fibres if they belong to the same motor unit. If the impulses carried by the motor unit reach the muscle fibre threshold then all muscle fibres attached to that motor neuron will contract 100%.
The number of muscle fibres in a given motor unit depends on the size, location, and function of the muscle.
Small muscle
One nerve fibre innervates a few muscle fibres. E.g., in an eye muscle, one motor neuron may innervate 23 muscle fibres.
Large muscle
One nerve fibre innervates more muscle fibres. E.g., in quadriceps, one motor neuron may innervate 500 muscle fibres.
All or none principle
Recall the all or none principle from the nervous system which states that the strength of a muscle fibre (or nerve cell) response is not dependent on the strength of the stimulus. I.e., as long as a stimulus is above a certain stimulus, the muscle will contract.
A motor unit will contract with maximum force if the stimulus is equal to, or above, the threshold
Typically, the different motor units of a muscle fibre are not recruited all at once or at the same time. While some contract, others relax, which delays muscle fatigue and allows whole-muscle contraction to be sustained over a longer period. In this model, the weakest motor units are recruited first with the stronger motor units are recruited if the task requires more force.
Excitatory thresholds
Different motor unit types have different excitatory thresholds. This is where muscle fibre types come into play, i.e., slow-twitch vs fast-twitch fibres. Have a look at the following graph.
As you can see in the graph, fast-twitch fibres have a higher threshold than intermediate and slow-twitch fibres. That means it takes more electricity to fire them. If the impulses sent down motor neurons to the muscle reached the threshold depicted in the graph, then only the slow-twitch and intermediate fibres would fire. It is important to note that slow-twitch fibres are innervated by slow-twitch motor neurons and fast-twitch fibres are innervated by fast-twitch motor neurons.
So if muscle fibres can only contract 100%, how can contraction strength vary?
Contraction strength
Contraction strength depends not on how strongly individual motor units contract but on how many motor units contract. When little force is needed, only a few motor units are stimulated to act. This indicates the principle of orderly recruitment.
Slow-twitch motor neurons are smaller and innervate fewer fibres than fast-twitch motor neurons. So, for low-intensity activity, most muscle force is generated by slow-twitch fibres. As intensity increases, intermediate fibres are recruited and at higher intensities, fast-twitch fibres are activated. The same pattern of recruitment occurs during events of a long duration.
For example, if weak contractions are sufficient to perform a task, slow oxidative fibres (SO) are recruited. If more force is needed, fast oxidative-glycolytic fibres (FOG) are recruited. If maximal force is required all fibres are called up (i.e., slow oxidative, fast oxidative-glycolytic and fast glycolytic fibres (FG)). Therefore, we can see, activating more muscle fibres produces more force. The nervous system recruits the number of motor units necessary to create the desired response.
Responses to Training
Let's start with a recap.
Recap
Individual muscles are a mixture of 3 types of muscle fibres (type 1, type 2a and 2b), but their proportions vary depending on the location and action of that muscle. But skeletal muscles only have one type of muscle fibre within a motor unit. This is demonstrated if we look at contractions.
- If a weak contraction is needed only the type 1 motor units will be recruited. These fibres are used mainly for endurance activities.
- If a stronger contraction is required the type 2a fibres will be recruited or used to assist the type 1 fibres.
- Maximal contractions facilitate the use of type 2b fibres which are always activated last. These fibres are used during ballistic or strength activities but tire easily.
Can you convert fibres?
In short, no. Traditionally, the total number of skeletal muscle fibres has been thought not to change.
While intermediate fibres will act like either slow-twitch or fast-twitch depending on the type of training undertaken, they do not convert. Similarly, slow-twitch fibres can not become fast-twitch and vice-versa.
The main reason why fibres do not convert appears to be because each type of fibre requires a specialised neuron to fire them, which cannot be changed.
Gender or age differences?
It is believed there are no sex or age differences in fibre distribution, however, relative fibre types vary considerably from muscle to muscle and person to person. Sedentary men and women (as well as young children) have approximately 45% type 2 and 55% type 1 fibres.
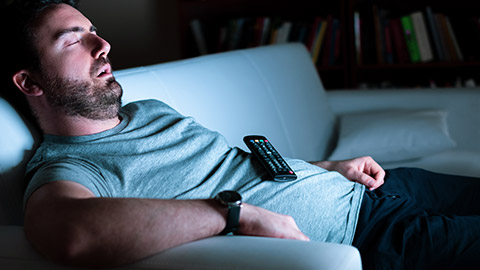
Fibre types and sport
People at the higher end of any sport tend to demonstrate significant variation in patterns of fibre distribution compared to the average population. For example, successful endurance athletes show a higher level of type 1 fibres. Successful sprint athletes, on the other hand, have large numbers of type 2b fibres in the key muscle groups.
Middle-distance event athletes show approximately equal distribution of the 2 types. This is also often the case for power athletes such as throwers and jumpers.
Fibre adaptation to exercise: Endurance athletes
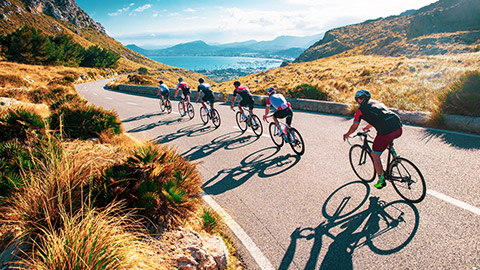
It has been well documented that elite endurance athletes possess a higher percentage of slow-twitch fibres in the muscles they use in their sport, compared to untrained individuals.
Is this due to genetics or years of rigorous training? The answer is difficult to get at directly because we don't have comparative muscle biopsies of great athletes before and after they started training and excelling in their sport.
However, good basic investigation using experimental models has helped generate some answers. Unfortunately, almost all of this research suggests it is impossible to change the fibre type, without changing the motor neuron type also….in short, this is not going to happen!
So, why bother training so hard?
Skeletal muscles do respond to chronic overload (training), by trying to minimise the cellular disturbance caused by the training. This means they adapt to prevent too much exercise stress from being put on the body.
With intense endurance training, fast-twitch fibres can develop more mitochondria and surrounding capillaries along with the slow fibres, so training improves the ability of your existing fibres to cope with the exercise stress you create for them.
Muscles are designed to produce force. Athletes train muscles to produce even more force in specific movements. A number of factors can have an impact on the ability of a muscle to produce force. Let's start with an obvious one.
Muscle size and force
Quite simply, a larger muscle has the potential to produce more force than a smaller muscle.
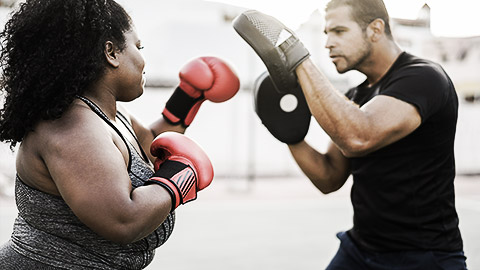
Keep in mind, a larger muscle doesn't always translate to better athletic performance. For example, throwing power, or a more powerful golf swing. A large set of biceps makes you better at bicep curls and filling out T-shirt sleeves! That said, if athletic form and function are well established, in some cases improving the size of key muscles will result in improved power output.
After hypertrophy training, you can expect to see adaptations in the muscles you have been targeting. Hypertrophy training targets mainly type 2 fibres as these are the biggest and produce the most force. We expect to see an increase in the size of the fibres due to an increase in the thickness of the sarcolemma. We also expect to see an increase in muscle strength due to a greater number of cross-bridges caused by an increase in sarcomeres in series.
Injury to muscle or connective tissue
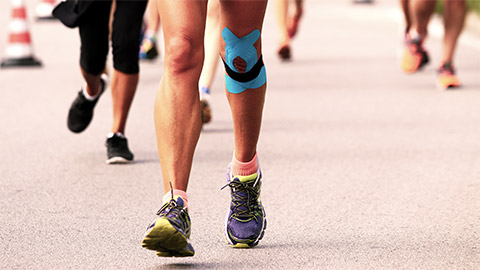
Damage to the muscle or connective tissue around a joint will lead to decreased force production of the muscles surrounding the joint. The brain is less likely to recruit musculature that causes pain. Trying to continue movement will most likely lead to movement compensation (e.g., limping).
The number and type of motor units recruited
The larger the number of motor units recruited, the greater the force produced.
Fast-twitch fibres produce more force. Muscle size is achieved through developing primarily fast-twitch fibres. Those muscles that have a large proportion of fast-twitch fibres will be stronger than other muscles. This is the main factor contributing to muscle force generation.
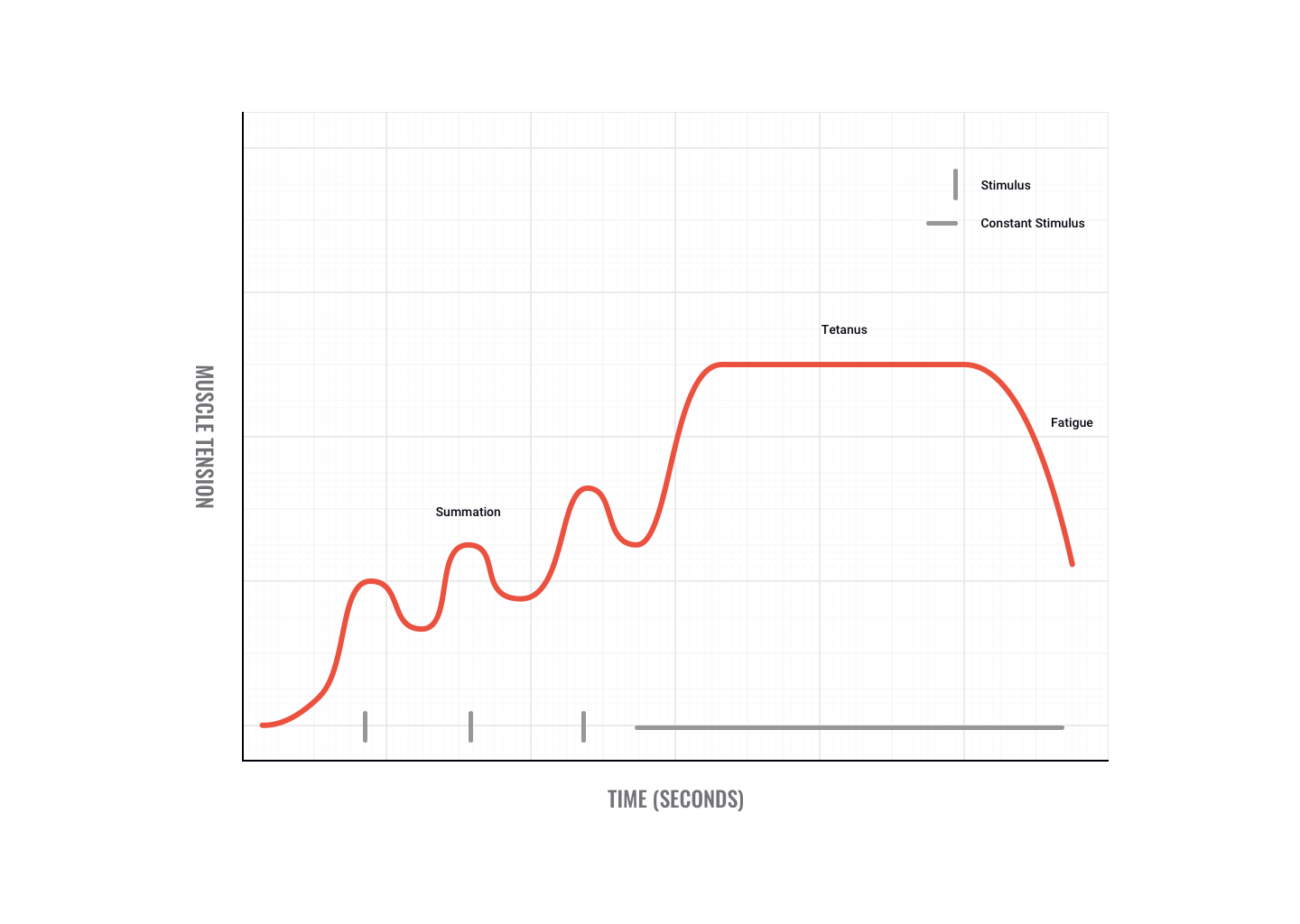
Tetanus
If motor impulses are sent fast and furious, twitches can add to produce a smooth, sustained, maximum contraction called tetanus.
Maximum tetanic contractions produce a force approximately 3 to 5 times a single twitch contraction.
An example of when we use tetanus is when trying to overcome a heavyweight. Initially, we may not be able to move the weight but as we send more motor signals we recruit greater numbers of motor units and finally overcome the weight, e.g. pushing a car or performing a heavy dead-lift.
Stretch: length-tension relationship
Optimal length - Lo
- Maximum number of cross-bridges
- No overlap of actin fibres from opposite ends of the sarcomere
- Optimal working muscle range from 80-120% of Lo.
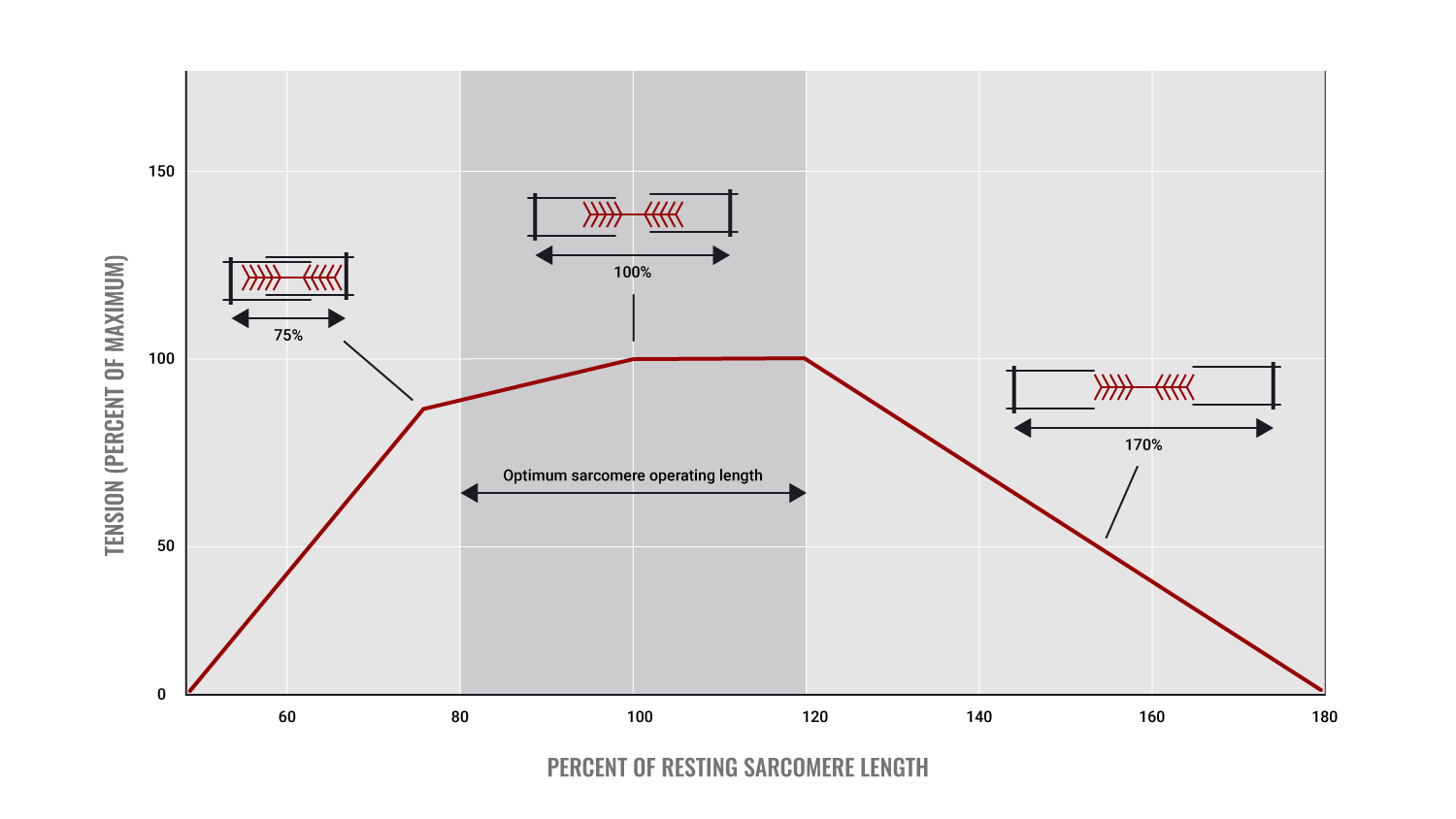
The starting length of a muscle before contraction
Starting length, more accurately referred to as "resting length" is where each muscle has an optimal length for efficient/ optimal muscle contraction.
This length allows for maximal cross-bridge interaction and will lead to the greatest muscle tension being produced.
The rule governing this is the length-tension relationship (also known as the 'force-length relationship'). This states that if a muscle is either over-stretched or over-shortened before the initiation of contraction, muscle force will be reduced. E.g., a 90° angle at the elbow joint is the ideal starting position for maximal force production by the biceps.
- Velocity of the Contraction
- Lighter loads can be moved faster
- Each muscle has its own optimal speed of contraction
- If OPTIMAL velocity is achieved - force is MAXIMISED
- If the velocity of contraction is too fast - force is reduced as there is not enough time for all fibres to form cross-bridges - i.e. slow-twitch fibres can't fire fast enough to aid contraction
- Optimal velocity will allow for progressive recruitment of fibres. Therefore greater numbers of motor units are activated leading to greater force production.
Velocity and power
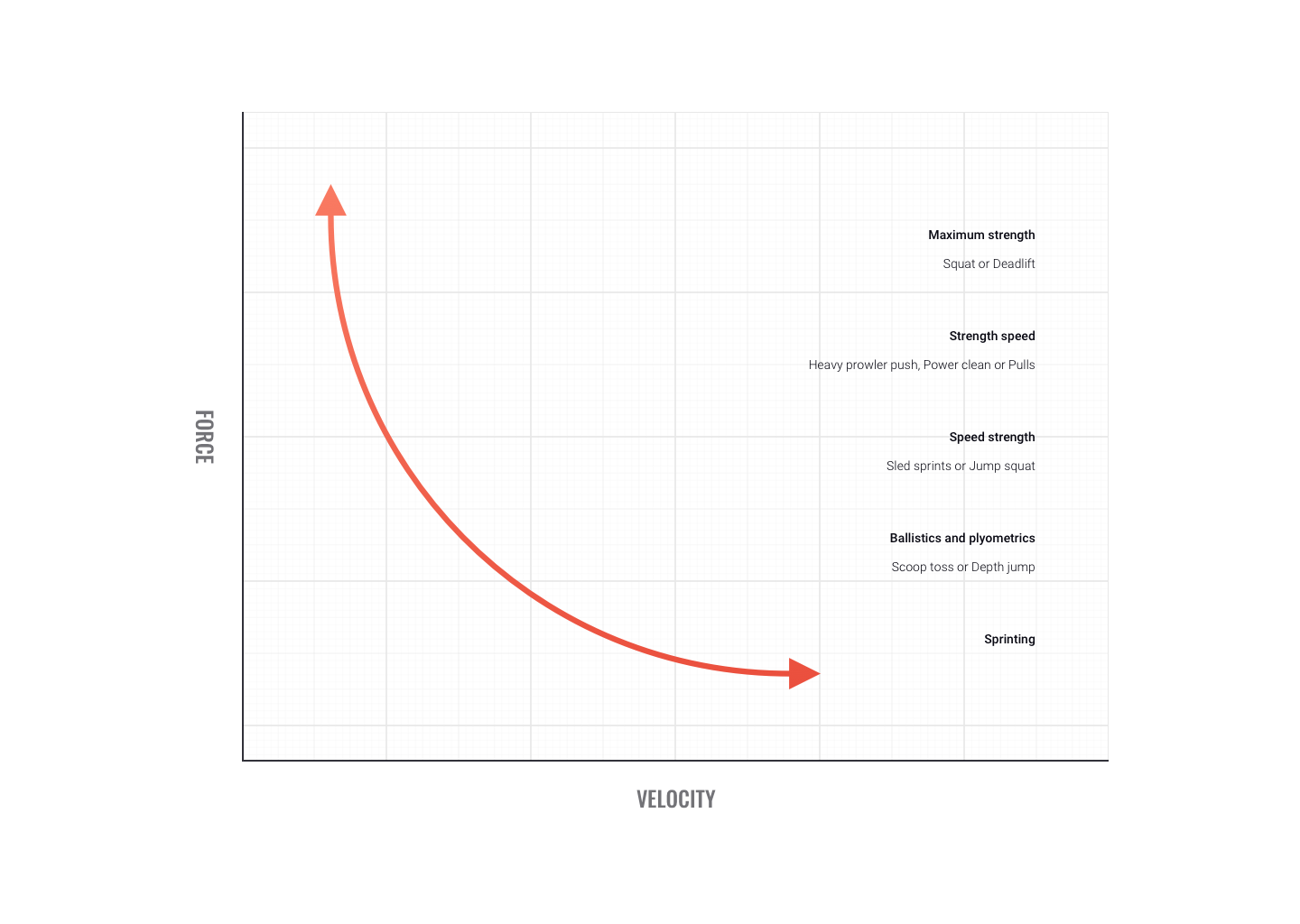
Some sports movements don't allow time for optimal force production. When force is produced quickly we term it 'power.
Power training targets fast-twitch fibres. While each muscle has its own optimal velocity for power production, on average around 300 degrees/sec produces peak power. At velocities faster than this, power appears to plateau.
Check that you have a good grasp of the muscular system before moving to the next topic. You'll be assessed on the muscular system in a combined test with energy systems.
Assessment prepararion
Congratulations for making it through the five body systems – skeletal & joints, nervous, cardiovascular, respiratory, and muscular. Now, take some time to recap and review the content up to this point, before moving onto the first assessment of this module.
Check back on the progression chart to make sure you are managing your time well and keeping up to date with your assessment hand ins. Head to the assessment tab to start your first assessment for this module. Ensure to watch the assessment overview video thoroughly and refer back to Ready, Set, Go! For any queries about downloading and uploading assessments to the platform.
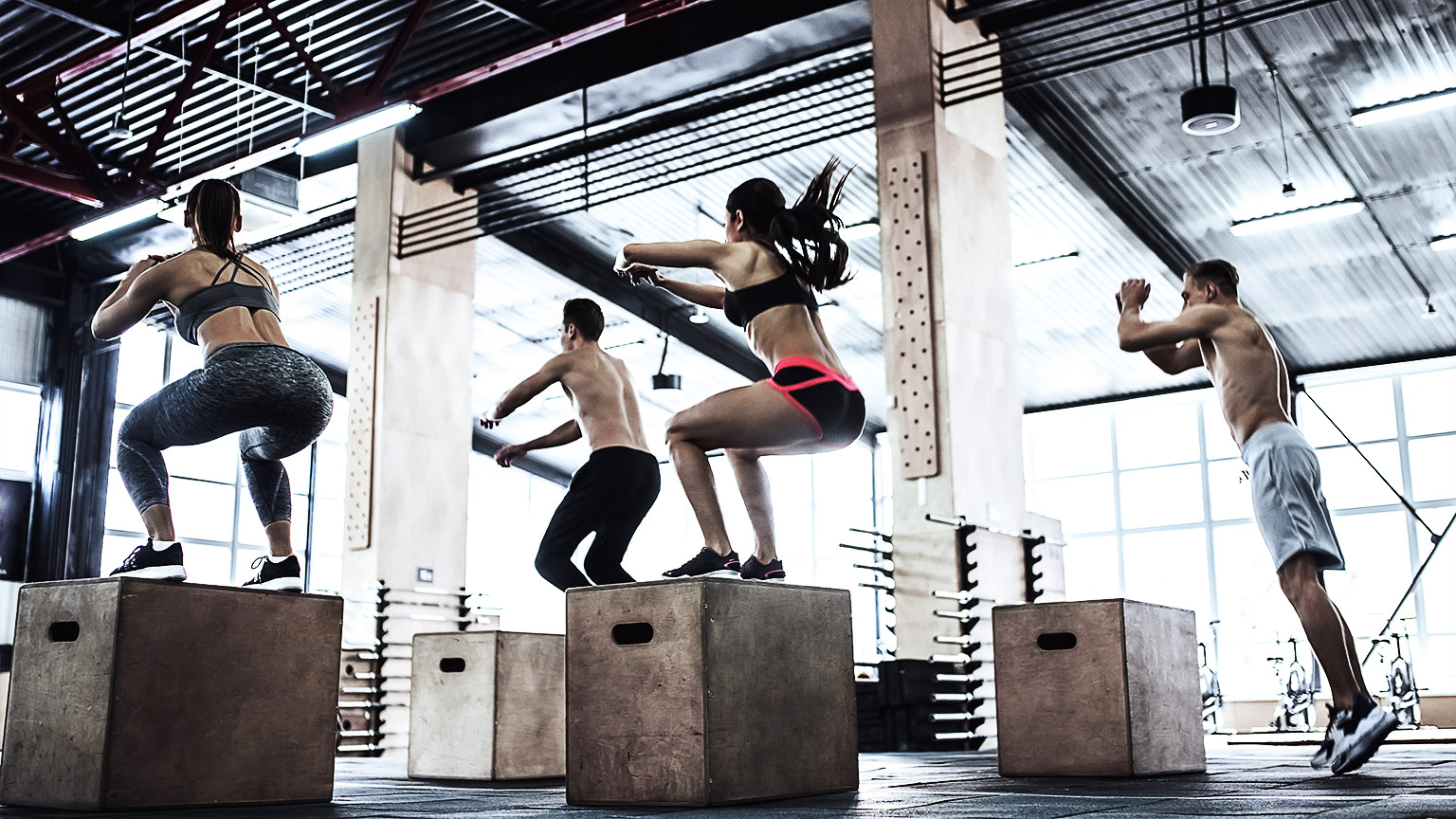