Introduction
(Video script)
“Welcome to the Muscular System! In this module we will explore key muscle terminology along with the structure and function of skeletal muscle. This includes getting to know muscle types, shapes and characteristics. We will learn about the ways in muscle contracts to move and hold us in place. From a more practical perspective you will learn the location and action of the main movement muscles we use during exercise and the exercises that target them in the gym.
Once we have learned the basics, we will begin to look at common muscle imbalances that we are likely to come across in a role in the sport, recreation and exercise industry. We will cover how they typically occur, how we can test to isolate common imbalances and how we go about correcting them.
Our online learning sessions will be used to recap the key learning we have completed in face-to face classes. Additionally, online sessions will be used to introduce the intricate inner workings of muscle, where we will explore the different micro-structures that make up a muscle, how they work together to contract muscle, and how exactly the nervous system selects and fires different muscle fibres for different actions.
The muscular system is a fascinating topic. We hope you enjoy learning how your muscles allow you to manipulate your environment, play sport and do all the other activities you enjoy.
Enjoy your third week of online learning.
There are three types of muscle tissue found in the human body. These are:
- Skeletal muscle
- Cardiac muscle; and
- Smooth muscle
Each of these muscle types have specific functions in the body. The muscle type we are most focused on in this topic is skeletal muscle. Skeletal muscle has four key properties associated with it. See what you can remember about the muscle types and the properties of skeletal muscle by answering the following questions.
H5P here
Muscles come in a wide variety of shapes and sizes. This allows for differences in the way their fibres are organised. The different shapes allow for different alignment of fibres (i.e., the direction that the fibres run). The way in which muscles attach to bone and where they attach to the bone can also influence the actions they cause. These differences allow the muscle to create a wide range of movements across the skeleton.
However, bones do far more than simply allow us to move. They have a range of functions that are important for maintaining homeostasis in the body.
The following task is designed to check your retention of content we have covered on the shapes, attachment and function of muscle. Feel free to read over the related notes in your learner resource before attempting these questions.
H5P here
All skeletal muscles attach to at least TWO (2) bones. At least one attachment is designed to act as an anchor (providing a base for the movement to be generated). The other attachment(s) points act to move the bone they are attached to (cause body movements).
Having an understanding of where muscles attach to bone can help you get a full picture of where muscles are located and the type of movement they can produce. The terms origin and insertion are used to indicate where muscles connect with bones.
The following task is designed to assess your understanding of muscle origin and insertion.
H5P here
Now that we have made a great start in our learning about muscle anatomy (the types, shapes, location and attachment of muscles), it’s time we learned about the inner workings of muscle. We know that muscles contract, but understanding how that process occurs, and the way in which the nervous system selects muscle fibres during movements, will go a long way to better understanding why we choose the exercise program variables we do to maximise our performance gains.
In this sub-topic we will begin to focus on the physiology (or function) of muscle, with a particular focus on the process of muscle contraction and muscle fibre recruitment. Before we dive into how a muscle contracts, we have to learn a little more muscle anatomy. You are about to be introduced to the star players in the muscle contraction team. They may be small, but they are mighty!
Muscle Micro-Anatomy
In order to understand the process of muscle contraction, we need to know the parts involved. Until this point, we have talked about muscles only in a macro sense (i.e., whole muscles). But contraction actually occurs at a microscopic level. To understand just how microscopic, we need to start with a whole muscle and work our way back to give this some context.
The image above shows a muscle connecting to a bone through a tendon. For ease of understanding, this muscle is a fusiform muscle with all fibres running in the same direction. The muscle has been cut through the widest portion of its belly. Inside, you can see that the fibres inside the muscle are organised into bundles. These are called fascicles. Depending on the size of the muscle, and the types of muscle fibres it contains, a fascicle could contain between 10-100 muscle fibres. The image shows a single fascicle extending from the muscle belly, with a single muscle fibre protruding from it.
All structures from the entire muscle to the single fibre are wrapped in a layer of connective tissue. These layers (the epimysium, perimysium and endomysium) enclose and separate the tissues within the muscle as well as contain blood vessels and nerves. The deeper layers also play a key role in the growth and development of muscle.
The Muscle Fibre
Let’s focus in on the single muscle fibre. To give the image above some context, a single muscle fibre is the width of a human hair! They are practically invisible to the naked eye. A muscle fibre can be as short as a few millimeters, or in the case of some of the fibres in our longer thigh muscles, over 35cm long.
But a muscle fibre is not the smallest component of a muscle, not even close. Inside a single muscle fibre are bundles of even smaller fibres that run the length of the fibre. These are known as myofibrils and are where the action of muscle contraction occurs.
Each muscle fibre can house several hundred to several thousand myofibrils. The image above shows the myofibrils within a muscle fibre. It also shows a single myofibril extending from the muscle fibre. You will note that myofibrils have a striped appearance. In fact, it is the myofibrils within skeletal muscle that give it its striped (striated) appearance. The stripes reflect the fact that there are even smaller components found within the myofibrils!
A myofibril is essentially a tiny cylindrical tube that is made up of repeating contractile sub-units called sarcomeres. These sarcomeres run the length of the myofibril and are where muscle contraction occurs.
The Sarcomere
If we can understand how one sarcomere contracts, we can then apply this knowledge to the remainder of the myofibril, the other myofibrils within the muscle fibre, and the other fibres that will also contract during a given movement. Each sarcomere contains two tiny myofilaments known as Myosin and Actin. These microscopic components are force generators within muscle.
The image above shows a single muscle fibre and the myofibrils within it. It also shows a single myofibril with its repeating sub-units (sarcomere). It then shows an enlarged view of the two myofilaments within a sarcomere. Myosin is the thicker myofilament (red), while Actin is the thinner myofilament (purple).
Watch
This video recaps on the key information we have covered so far. It also introduces a few other key structures that we need to be aware of before we can discuss the process of muscle contraction. Listen out for these as you watch.
- Video Title: Structure of Skeletal Muscle Explained in Simple Terms
- Watch Time: 2:10
- Video Summary: In this video the structure of skeletal muscle explained from the whole muscle down to actin and myosin.
- Pre Watch Question: Listen out for a couple of new structures that are introduced during the video.
The Sarcolemma and Sarcoplasm
Each muscle fibre is surrounded by a plasma membrane called the sarcolemma. This outer layer of the muscle fibre allows attachment points for neurons and helps to conduct electrical signals across the muscle fibre. The sarcolemma also plays a critical role in muscle development and training induced growth which we will discuss later in the topic.
The sarcoplasm is a water-based jelly like substance that surrounds the myofibrils within a fibre. It contains energy in the form of ATP and glycogen (glucose) that fuel the muscle fibre contraction. It also contains a protein called myoglobin which has the ability to store oxygen molecules. In short, the sarcoplasm has everything the muscle fibre needs to perform contraction.
The Sarcoplasmic Reticulum
The sarcoplasmic reticulum is a network of tubes and tubules (smaller tubes) that sit just under the sarcolemma and surround the myofibrils in a muscle fibre. This structure plays a key role in muscle contraction. It stores calcium, and when needed releases it to be used in the process of muscle contraction (more on calcium’s role in this soon). The sarcoplasmic reticulum can be seen in the image below (in blue).
Pause and Recap
You have just been introduced to a lot of new information (as well as some pretty tricky words). We now know all the players involved in the muscle contraction process. But before we dive into that process, let’s do a little recap activity on the micro-anatomy of muscle, so you are comfortable with all of them (before we find out what they do).
The following video will recap the structures that you have been introduced to in the learning above. The video will stop at intervals and ask a question that checks your understanding of the learning so far.
The Sliding Filament Theory of Muscle Contraction
Now that you are fully aware of the components that make up a muscle, we can start to look at how a muscle contraction occurs. As we know from our previous learning, muscle contractions are stimulated by electrical impulses travelling through motor neurons. These motor neurons arrive at neuromuscular junctions (connection points between the end of the motor neuron and the sarcolemma of the muscle fibre).
The image below shows how a motor neurons synaptic endings branch out to connect with muscle fibres forming these junctions.
A series of actions occur at this meeting point (that are not important for you to know). The end result is that electrical impulses are passed to the sarcolemma and sarcoplasmic reticulum of the muscle fibre, where they are spread throughout the muscle fibre. This is what starts the muscle contraction process.
Myosin and Actin
As we learned earlier, myosin and actin are the key structures that create muscle contraction within the myofibril. The thicker myosin filament is a protein structure that contains several hundred globular heads, which protrude from its main structure. These myosin heads form connections called cross-bridges with the thinner actin filaments that wrap around myosin. You can see this relationship on the image below. The actin filaments are pink, the myosin filaments are brown with orange myosin heads. In this image the myofilaments are at rest (no contraction occurring).
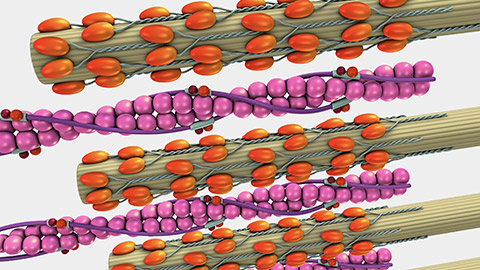
Myosin is highly attracted to actin (it wants to connect with it). Myosin’s job is to reach up with its little heads, grab onto actin and pull the actin strands towards the centre of the sarcomere (causing contraction of the myofibril). But in its resting state the binding sites on actin that myosin can connect with are blocked by a barrier. This barrier is known as tropomyosin.
Tropomyosin and Troponin
Tropomyosin is a regulatory protein strand that wraps around the actin myofilament and blocks the myosin head binding sites. This barrier protein is regulated by another protein structure called troponin. Troponin is like the “gate keeper”. The image below shows a single strand of actin. You can see these two protein structures labeled on the image.
In order for a muscle contraction to occur, troponin must “lift” tropomyosin off the active binding sites, so that the myosin heads can reach up and pull on actin (causing contraction), but what makes troponin lift tropomyosin? You guessed it……calcium!
The Role of Calcium in Muscle Contraction
Nerve impulses travel down motor neurons and stimulate the sarcoplasmic reticulum to release its store of calcium. Calcium binds to troponin (the gate keeper) and the subsequent reaction causes troponin to rotate tropomyosin (the barrier), lifting it off the myosin binding sites. Myosin heads are attracted to the exposed binding sites, so they reach up and connect with actin, they then pull actin towards the centre of the sarcomere (from both ends).
The forming of a cross bridges between myosin and actin can be seen on the image below.
This pulling action is known as a power stroke. When the myosin head has performed one power stroke, it disconnects from actin, rotates back and reconnects to perform another power stroke. Approximately 3-5 power strokes can be performed by each myosin head a second.
The actin myofilaments are connected to the Z-line of the sarcomere. When actin is pulled towards the centre of the sarcomere, the sarcomere shortens. This can be seen in the image below.
This same shortening process occurs in every sarcomere, in every myofibril, in every muscle fibre stimulated by the connecting motor neurons, leading to muscle contraction.
The Role of ATP
Adenosine Triphosphate (ATP) is the base unit of energy in the body. As we learned earlier, it is stored within the sarcoplasm of a muscle fibre and works with oxygen to produce energy. ATP fuels the muscle contraction at the myofilament level. When calcium binds to troponin to remove tropomyosin from the binding sites on actin, ATP binds to the myosin heads providing the energy they need to reach up, grab actin and perform a power stroke. Another molecule of ATP binds to the myosin head to break the crossbridge connection and rotate the myosin head back into position to perform another power stroke. The process is repeated until the contraction is complete.
You can see this process occurring in the image below.
When we talk about using up energy during exercise (or burning calories during exercise), this is where it happens! The more muscles we use, the more cross bridges and power strokes are being performed in muscles, which leads to greater energy consumption.
The Importance of Calcium and ATP
Muscle contraction can continue as long as calcium and ATP are present in the muscle. Of course, we have a substantial deposit of calcium in our bones that we can call on, but if it runs out “on-site” their will of course be issues with contraction as troponin will no longer be able to keep tropomyosin off the binding sites.
ATP depletion in muscle fibres is the primary cause of muscle fatigue. While ATP can be made from fuel sources such as glucose, protein and fat, if the demand for ATP by muscle fibres outweighs the ATP stores in muscle (and the ability of the cells to produce new ATP), then we will be unable to perform power strokes and the muscle cease to be able to produce force.
How a Contraction Ends
If we choose to end a muscle contraction, inhibitory impulses are sent along the motor neuron stimulating the sarcoplasmic reticulum to take any remaining calcium back into storage. This means tropomyosin returns to cover the binding sites on actin, so myosin heads can no longer perform power strokes. A final molecule of ATP is used to break any connected myosin heads away from actin and the muscle fibre relaxes.
Mind blown yet? Wait until you see this process in action!
Watch
The video below recaps the key muscle structures involved in contraction as well as the steps that lead to muscle contraction (the sliding filament theory). Enjoy!
- Video Title: Sliding Filament Theory of Muscle Contraction Explained
- Watch Time: 2:20
- Video Summary: This video explains how muscles contract at a cellular level
- Pre Watch Question: See how many of the structures that we have discussed you can identify during the video.
Recap of Learning So Far
Let’s see how well you have retained what you have learned in this lesson so far. The task below is a series of questions to check your understanding of the process of muscle contraction.
H5P here
During movements, muscle fibres need to be recruited and contracted in a specific sequence. As previously discussed in earlier learning, this process is governed by the nervous system (i.e., frontal lobe primary motor cortex and cerebellum). Before we can discuss what muscle fibre recruitment looks like from a muscle perspective we have to understand a couple of key pieces of information.
- Muscle fibres cannot be contracted individually - One motor neuron innervates multiple fibres
- Muscle fibres can only be contracted 100% - It’s all or nothing!
- Different muscle fibre types have different stimulus thresholds
Motor Units
One neuron innervates multiple muscle fibres. That means when electrical impulses are sent down motor neurons, all the muscle fibres that connect to the neuron will contract. One motor neuron and the muscle fibres it innervates is known as a motor unit. For this reason, we will now no longer refer to muscle fibres contracting, but instead will use the term “motor units”.
One motor unit
The number of muscle fibres in a motor unit depends on a couple of key factors including:
- The size of the muscle: In small muscles one motor neuron might only innervate a small number of fibres. For example, the motor neuron that innervates the muscle that constrict the pupil of the eye only innervates 23 muscle fibres. In larger muscles like the quadriceps, one motor neuron may innervate around 500 fibres.
- The function (and location) of the muscle: Motor units in the legs and back tend to be large, with thousands of muscle fibres per motor neuron. This is because these muscles are used for more powerful, but less coordinated actions than say muscles in the hands, which have an average motor unit size of 10-100 fibres allowing them to manipulate our environment with fine, coordinated control (Cech & Martin, 2012). This also means the leg muscles have less efficiency than some other muscles as they fire thousands of fibres during even small movements. For example, the calves are said to have a motor unit ratio of 1 motor neuron to every 2000 fibres. This may explain why the calves are often the first muscle to fatigue during ongoing full body exercise.
The All or Nothing Principle
Motor units cannot partially contract. If a motor neuron stimulates the fibres in a motor unit to contract, they will all contract with 100% of their force.
The All or nothing principle states that:
A motor unit will contract with maximum force if the stimulus is equal to, or above, the threshold of the motor unit.
So, if motor units can only contract with 100% force, how do we perform partial movements, or gentle contractions?
Easy. We only select the number of motor units we need to get the job done.
Thresholds
While we won’t cover these in detail until later in the topic, you are probably already aware that your muscles are made up of different fibre types (i.e., slow twitch, intermediate and fast twitch). These different muscle fibre types have different excitatory threshold levels (i.e., the required electrical stimulus needed for them to contract).
You can see in the image below that slow twitch fibres (which tend to be the smallest and weakest) have the lowest thresholds, followed by intermediate and then fast twitch fibres. If this level of electrical stimulus was to be sent to a muscle, only the slow twitch and intermediate fibres would contract (as the threshold of the fast twitch fibres has not been reached.
This means that the electrical stimulus required to contract slow twitch motor units, is less than what is required to innervate fast twitch fibres. The brain is able to send electrical impulses of graded intensity along motor neurons that will cause some motor units to contract, but not others. This is critical to allow us to moderate the strength of muscle contractions for the different activities we do.
Without these differing thresholds, even simple tasks would be fatiguing. For example, imagine if every motor unit in your bicep contracted fully every time you raised your coffee to your lips (essentially performing a maximal bicep curl each sip), the muscles of your arm would start to burn and fatigue well before you finished the cup!
The brain loves efficiency, so it will always try to perform an activity with the least number of motor units firing as possible. This ensures that our muscle don’t fatigue quickly.
Not only does the brain choose the least number of motor units possible for a task, but it also recruits the weakest motor units first, only calling on stronger motor units if the initial motor units recruited can’t get the job done. This model of recruitment is known as the Principle of Orderly Recruitment.
Contraction Strength
Contraction strength does not depend on how strongly individual motor units’ contract but how many motor units’ contract. When only a little force is needed, only a few motor units are stimulated to act. The image below shows a simple representation of this. If this fascicle of fibres was only needed to produce a low force contraction, then the impulses sent down the motor neurons would only be at an intensity that would meet the slow twitch motor unit threshold. If a maximum contraction was required, then impulses would need to reach the threshold of the fast twitch motor unit. That way all the fibres attached to these motor neurons would contract leading to a stronger contraction.
You can see this principle of orderly recruitment in action for yourself by performing the following simple task.
- Sit yourself at a table or desk.
- Allow your right arm to hang loosely at your side.
- Gently palpate (squeeze) your right arm bicep with the fingers and thumb of your left hand noting how “squishy” the muscle fibres are at rest.
- Perform an easy bicep curl (with no resistance) while continuing to palpate the bicep with your left hand. You should feel a slight firming in the biceps as some weaker motor units are recruited.
- Next, place your right-hand palm up flat against the underside of the table or desk and push up against the underside of the table. Continue to palpate your bicep and note how the bicep is becoming firmer as more motor units are recruited.
- Finally, increase the force of the contraction against the table. Your nervous system will continually recruit more and more motor units (and your bicep will get firmer) until one of two things happen (you lift the table, or your bicep starts to fatigue, and you decide to abandon the contraction).
Speed of Contraction
The speed that a contraction is performed at can alter the principle of orderly recruitment. The terms “slow and fast twitch” indicate how quickly different muscle fibres can be recruited. If a contraction is performed very quickly, slow twitch fibres might not have time to recruit, so the resulting force production will be less (as you would not be using all of your fibres).
This is why it is important to perform maximal strength activities at a steady pace (e.g., maximum deadlift, or pushing a van). If you were to try and attack the movement too quickly, you would bypass your slow twitch fibres leading to quick failure.
We will discuss more relating to this concept when we cover muscle fibre types in class soon.
Recap of Key Learning
Watch
The following video recaps the key learning we have done on motor unit recruitment. Use it as a means of embedding this important contend. A practical task and series of recap questions will follow the video.
- Video Title: Recruitment of Small and Large Motor units
- Watch Time: 3:09
- Video Summary: This video explains how the brain can adapt the strength of muscle contraction to meet the demands of a task.
- Post Watch Task: A series of recap questions will follow this video.
H5P here
Exercises can be classified by the number of joints (and therefore muscles) that are engaged during the exercise. Exercises that use multiple joints are known as compound exercises, while those that only use one joint are called isolation exercises.
Even when an exercise only uses one joint, there can still be a number of muscles involved in the movement. There are the obvious ones that provide the majority of the movement force, others that help them out, and even more that have the role of stabilising the limbs or torso to ensure the movement is efficient and that energy is not wasted correcting for unwanted movements.
There are also muscles, who’s role it is to provide a resistive force against the prime mover muscle. But this doesn’t occur for every movement.
The following questions are designed to assess your retention of the content related to exercise classification and the roles of different muscles in movement. Feel free to read over your course notes before attempting these questions.
H5P here
If you wish to work in certain frontline roles within the sports and fitness industry, it is vital that you know what muscles are targeted in common gym -based exercises. This information will also make it easier for you to succeed in your upcoming exercise prescription modules.
When writing balanced programs, it is not only important that you can identify the agonist muscles of an exercise, but also that you have the ability to identify opposing exercises that will ensure the antagonists of those muscles are also targeted equally. This helps to avoid creating a muscle imbalance.
The following task is designed to test your ability to identify the muscles that are targeted in a range of common exercises and your ability to suggest opposing exercises to target the antagonists of these muscles. Complete the tasks by dragging the muscle names into the appropriate spaces in the statements to follow.
H5P here
There are three main contraction types we perform during exercise. These are:
- Isotonic Eccentric contractions – when a lengthens while producing force (under control)
- Isotonic Concentric contractions – when a muscle shortens while producing force
- Isometric contractions – when a muscle produces force but doesn’t change length
Many people think that both the agonist and antagonist are targeted during a weighted exercise. That is not the case. The same muscles (agonists and synergists) are worked in both the up and down phases of a lift (and both phases are critical to developing strength).
This series of questions is designed to check your ability to identify the different types of contraction in common gym exercises. The exercise images shown in these questions will come with arrows which alert you to the phase of the lift that is being performed.
H5P here
Human muscle tissue is made up of three predominant fibre types. They are classified into different types based on their physiological and biochemical properties, including the speed of contraction, resistance to fatigue, and energy production pathways. For those looking to work in the SRE industry training others, it is important to have an understanding of fibre types to appreciate how the body responds to different forms of exercise and training.
In this subtopic we will cover:
- The three main fibre types, their defining characteristics and function in SRE.
- The role of genetics in muscle fibre allocation and what this means for you.
- How exercise and training can adapt muscle fibre characteristics and performance.
- That factors that affect muscle fibre force production
- The three main muscle fibre types found in human muscle tissue are:
- Slow-Twitch (Type I) Muscle Fibres
- Fast-Twitch (Type II) Muscle Fibres, including:
- Type IIa - Intermediate fibres
- Type IIx - Pure Fast-Twitch fibres (sometimes referred to as type IIb fibres in the literature)
Each fibre type has unique characteristics and functions, which are optimised for specific types of physical activities.
Some Key Terms
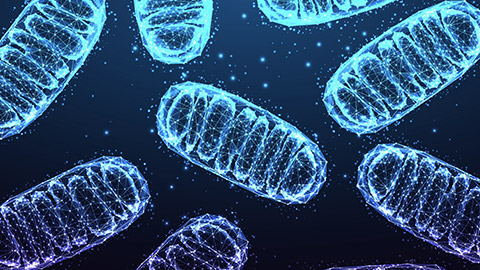
Mitochondria: The powerhouse of a cell. The mitochondria of muscle fibres produce the energy that the fibres need to contract. They take nutrients stored in the muscle (like glucose, fat and amino acids) and along with oxygen, convert them into ATP which fuels the myosin head action in muscle contraction. Essentially, the more mitochondria a muscle cell has, the better it can sustain activity without succumbing to fatigue.
Myoglobin: Myoglobin is a red pigment which acts as a storage site for oxygen within the muscle. It takes the oxygen brought to the skeletal muscle fibres by blood (hemoglobin) and stores it for use in the muscle fibre. This oxygen is then used alongside nutrients like glucose to produce ATP in the muscle fibre. The more muscle fibres a muscle fibre has, the redder it presents in colour. More on this soon.
Some Overriding Concepts
Before we dive deeper into the different muscle fibre types, there are some key concepts that you need to understand about muscle function.
First, in order to continue contracting a muscle requires a steady supply of oxygen and fuel. This ensures the production of ATP keeps up with muscle demand (i.e., the fuelling of cross bridges and power strokes between actin and myosin. As we learned in earlier content, if there isn’t enough ATP available, muscle contraction simply can’t occur.
Next, you need to have a clear understanding of the difference between aerobic and anaerobic muscle respiration. The term ‘aerobic’ respiration refers to the process by which muscle fibres produce ATP when oxygen is readily available. This is typically seen in lower intensity activity where the blood supply can keep supplying the working muscles with oxygen at the rate the fibres need it. This allows the mitochondria to generate a constant supply of ATP for muscle contraction to continue at the same rate.
The term ‘anaerobic’ respiration refers to the process by which muscle fibres produce ATP when oxygen isn’t readily available. This situation arises when the muscle fibres are working at high intensities and the blood supply isn’t able to deliver oxygen to the muscles at the rate the muscle fibres require. In this case, the muscles produce ATP by breaking down glucose in a quicker, less efficient process. This produces a lot of waste product (lactic acid) which leads to rapid muscle fatigue.
The difference in these energy production pathways can be seen in the image below.
Characteristics of Different Muscle Fibre Types
Now that we know these additional components and concepts related to muscle function we can discuss the differences between muscle fibre types.
H5P here
Summary of Muscle Fibre Characteristics
Structural Characteristics | Type 1 – Slow Twitch | Type IIa - Intermediate | Type IIx – Fast Twitch |
---|---|---|---|
Mitochondria Level | MANY | MANY | FEW |
Capillary Density | MANY | MANY | FEW |
Myoglobin Content | HIGH | MODERATE | LOW |
Colour | RED | RED/PINK | PALE PINK/WHITE |
Functional Characteristics | |||
Energy System Used | AEROBIC | BOTH AEROBIC AND ANAEROBIC | ANAEROBIC |
Fatigue Rate | SLOW | SLOW/MODERATE | FAST |
Contraction Speed | SLOW | FAST | FASTEST |
Force Production | LOW | HIGH | HIGHEST |
Size | SMALLEST | MODERATE | LARGEST |
Motor unit size | SMALL | MEDIUM | VERY LARGE |
Order recruited in activity | FIRST (Unless explosive contraction) | SECOND | THIRD |
Activities used in | Endurance, posture, longer duration/lower intensity | Endurance and longer duration high intensity. | Short duration, explosive activities. |
Let’s see what you have been able to retain about muscle fibre types.
H5P here
Fibre Distribution
Humans have a mix of muscle fibres throughout their body. In the general untrained population, it has been suggested that muscle fibre distribution is approximately 50/50 slow twitch and fast twitch. The majority of fast twitch fibres are thought to be type IIa intermediate fibres, with the smallest proportion of fibres being pure fast twitch.
However, the ratio of muscle fibre types in human skeletal muscle varies widely between individuals (and muscles). Fuku et al (2019) collated studies to date on muscle fibre type allocation in humans and found that fibre allocation ranges were as follows:
- Slow twitch – between 15-85% of total fibres.
- Type IIa fibres – between 5-77% of total fibres.
- Type IIx fibres – between 0-44% of total fibres.
Studies have also shown that certain muscles of the body exhibit high levels of certain fibre types in all humans (based on their function). For example, the extraocular muscles of the eye have a high proportion of fast twitch fibres in all humans, so the eye can react to fast moving stimuli. The soleus muscle in the calf typically exhibits high levels of slow twitch fibres to allow it to withstand fatigue in its key role of posture (when standing).
The Colour of Muscle
Human muscles are typically a mix of muscle fibre types, but as you can see from the wide variance in fibre distribution discussed above, the composition of an individual muscle can vary greatly.
The distribution of muscle fibre types in a muscle will influence its colour. Let’s take a moment to consider the colour of muscle tissue. Luckily, we don’t often see human muscle tissue (in the flesh). However, we can better understand the influence of muscle fibre type on muscle tissue colour by considering what we already know about the muscle tissue of other animals (that we do see regularly).
Beef, Pork or Chicken
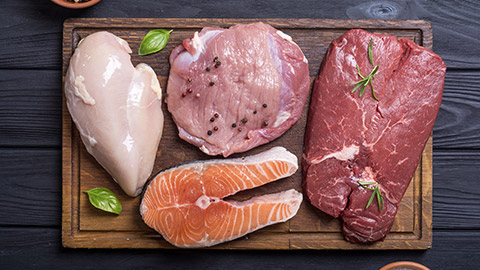
Have you ever thought why some cuts of meat are dark and some cuts are lighter? For example, chicken vs beef. Think about what you now know about the characteristics of the different fibre types.
The answer to this question revolves around the amount of blood and myoglobin in a muscle. Slow-twitch fibres have more capillaries (and therefore blood) and higher levels of myoglobin (a red pigment). Therefore, muscles composed of slow-twitch fibres are red in nature. Fast-twitch fibres have fewer capillaries and less myoglobin. Therefore, muscles made up of mainly fast-twitch fibres are lighter in colour.
Now consider for a moment what a cow does all day? It stands and eats. Cows are predominantly slow-twitch orientated animals (have you ever seen a cow jump or sprint?). Beef is very red in nature as it is full of blood and myoglobin.
Chicken: Light and Dark Meat
Source: https://nz.pinterest.com/pin/100557004160711664/
Chicken is an interesting example. Breast and wing meat is very light in colour, whereas leg meat is darker. This makes sense when you think about how these areas are used. Breast meat is obviously attached to wings, which chickens use to quickly fly away when spooked (fast-twitch orientated), whereas legs are for walking and roosting (slow-twitch orientated) and therefore have slow twitch fibres (and thus) blood within.
What About Us?
Humans also have darker and lighter meat. Some of our muscles, like postural muscles in the lower leg and neck are almost all slow-twitch fibres. Others such as those controlling eye movements are made up of only fast-twitch fibres.
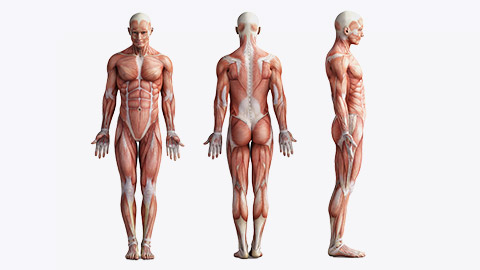
The majority of human muscles contain a mixture of both slow and fast fibre types, giving them more of a pink colour, but as we have already established above, the colour of one human’s muscle to another could be drastically different!
The wide ranges of fibre distribution discussed earlier, indicate that humans can exhibit huge variance in muscle fibre types. Let’s explore why.
Genetics
Genetics plays a significant role in determining the proportion and distribution of muscle fibre types in an individual’s body. The type and allocation of Type I (slow-twitch) and Type II (fast-twitch) muscle fibres a person has is largely inherited, meaning that you are born with a certain ratio of these fibres that remains relatively constant throughout life.
These are two very different animals (in terms of muscle)
Studies have clearly shown that athletes competing at the highest level in their sports are not average in any respect when it comes to their muscle fibre allocations.
Power and speed athletes tend to have much higher proportions of fast twitch muscle fibres (sometimes as high as 80%). Distribution of fast twitch fibres in the key muscle groups (glutes, quads and calves) can reach as high as 90%).
Conversely, elite endurance athletes like marathon runners exhibit a much higher proportion of slow twitch muscle fibres (sometimes as high as 90%).
Unfortunately, for most mere mortals with distributions close to 50/50 slow and fast twitch fibres, it is not a level playing field and certainly not one they could ever compete in at an elite level.
Unfortunately, science suggests you cannot significantly alter your fibre allocation. There is no evidence to date that suggests you can convert slow twitch fibres into fast twitch (and vice versa). Therefore, you can largely thank (or blame) your parents for your level of athleticism or sporting success or failure.
So, should I even bother training if I don’t have the right genetics?
Yes! While training will not fundamentally alter your genetic make-up, training can influence the performance characteristics of muscle fibres (such as enhancing endurance in fast-twitch fibres). While we are all limited by the genetics we have, skeletal muscle will still respond to training, so all of us can benefit from muscle adaptations leading to increased performance. Rather than converting fibres, training optimises the fibres you already have to assist the training you do.
Let’s explore the adaptations that occur in muscle following a period of specific training.
Muscle Fibre Adaptation from Endurance Training
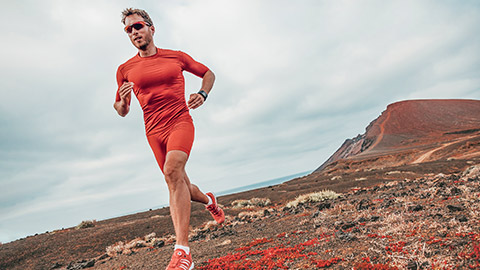
After a quality training block focusing on muscular endurance, all fibre types will exhibit an increase aerobic capacity due to noticeable increases in mitochondria (size and number) , myoglobin and capillary density, making them more fatigue-resistant during endurance activities as they become better at generating ATP over long periods and can deliver oxygen and fuel more efficiently to the fibres. Endurance training also leads to an increase in glycogen storage capacity (glucose) in muscle along with an increased efficiency in the fibres ability to burn fat as a fuel source. These changes appear mainly in type 1 and type IIa fibres. Type IIx fibres will also start to act a more like type IIa fibres, exhibiting many of the same changes but to a lesser degree.
Muscle Fibre Adaptation from Strength, Power and Speed Training
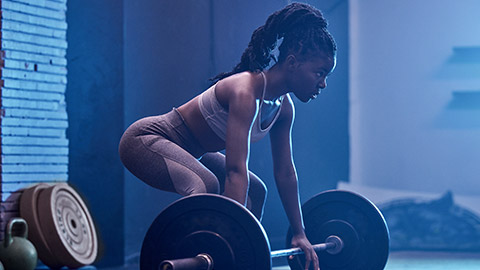
Fast-twitch fibres (Type IIa and Type IIx) are responsible for explosive and powerful movements. These fibres increase in size (hypertrophy) due to the high demands placed on them during power, speed and strength training. Type IIa fibres become more prominent as they adapt to both the speed and slight endurance demands of speed training, whereas Type IIx fibres would increase in both size and power output.
The growth of these fibres (hypertrophy) leads to increased muscle mass. Let’s take a quick look at the structural adaptations that cause hypertrophy.
The Mechanism of Hypertrophy
Schoenfeld (2021) defines muscle hypertrophy as an increase in the size of muscle tissue due to enlargement of the both the contractile elements of muscle, the expansion of the extracellular matrix of muscle to support growth and an increase in fluid within the muscle. This means that enlargement occurs to both the parts of the muscle that cause contraction and the layers of supportive tissue that surround them.
It is thought that hypertrophy of fibres is partly explained by an increase of sarcomeres (either in a parallel fashion or stacked on top of each other) within the myofibrils of fibres. This means more cross bridges forming inside fibres adding both size and strength.
Schoenfeld (2021)
The other factor that appears to enlarge muscle tissue during this form of training is ‘sarcoplasmic hypertrophy”. Essentially, the physical parts of muscle tissue (e.g., collagen) thicken and retain more fluid. This process is thought to happen in response to micro-damage caused through training which leads to repeated tearing and repair of the sarcolemma of the muscle fibre. In simple terms, if untrained muscle fibres are the diameter of a human hair, a bodybuilder or power trained athletes muscle fibres might be the diameter of uncooked spaghetti!.
Summary of Muscular adaptation
Once again, you can see how adaptable the human body is! The type of training you do, leads to adaptations that lead to increased muscular efficiency (for endurance), or improved force output for performance in strength or power pursuits. However, muscle does appear to be a system where adaptation has its limits.
While training can enhance muscle function and fibre efficiency, genetics largely determines your muscle fibre composition. This genetic foundation influences athletic potential in various sports and explains why some individuals are naturally inclined toward endurance activities, while others excel in power and speed sports.
While type IIa muscle fibres appear to be the most adaptable, taking on characteristics of other muscle fibres, it appears that any significant “switching” between Type I and Type II fibres is unlikely. This could be due to the fact that these fibres are innervated by different types of neurons. The slow twitch neurons appear incapable of sending impulses at the firing rates and neural drive required to contribute to fast twitch orientated activity.
So, Are Elite Athletes Born or Made?
It would appear the answer to this question is……both! Having the right genetic material is an obvious advantage when it comes to excelling in a particular field of exercise, however, the incredibly fine margins that exist at the top level suggest those who train the hardest (and smartest), have mental toughness and motivation to train daily are the ones who maximise their achievement. There are plenty of athletes with fantastic genetics that don’t make it to the top due to deficiencies in other areas.
What we do know, is that a person with standard genetic muscle material (50/50 ratio) could not compete with someone with superior genetic material under the same training conditions. Additionally, an athlete who competes at the elite level in one discipline (e.g., sprinting), would not be able to transition successfully to compete at the elite level in a disciple requiring a different genetic make-up (e.g., marathon). Athletes at the elite level in endurance are simply different genetic species than those who are breaking 10 seconds over 100m or throwing Javelins the length of a stadium.
Just Out of Interest
Ever worked out to the point where your muscles just won’t work anymore?
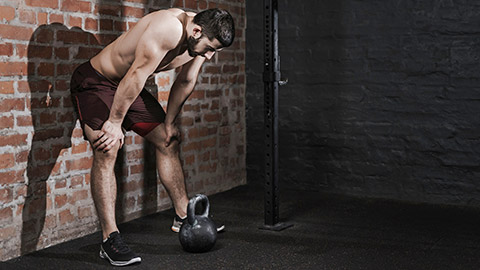
The term muscle fatigue is talked about often in SRE circles. But what is fatigue? Many believe it is simply a case of the muscles running out of fuel, but research has shown that even following exhaustive training, muscles still seem to have energy in reserve.
Others believe it is the inability of the body to deliver oxygen to the muscle or remove exercise wastes. There is certainly evidence to suggest this is part of the reason. But there also seems to be a nervous system component to fatigue that many people are not aware of.
Watch
The video below explains how biochemical nervous system fatigue occurs and how this alters the muscle’s ability to contract. There are a few new biochemical players introduced in this video. Don’t worry, you don’t have to remember these! But now that you have covered content on both the nervous system and muscular system inner workings, you are in a position to better understand this process in full. Enjoy!
- Video Title: The Surprising Reason our Muscles Get Tired
- Watch Time: 4:24
- Video Summary: This video explores why our muscles fatigue during exercise, how our muscles function, and how you can exercise longer without experiencing muscle fatigue.
Source: YouTube
H5P here
Muscle undergoes a series of changes across the human lifecycle. These changes can be broadly categorised into four life-stages:
- Childhood
- Adolescence
- Adulthood
- Older Age
The following questions are designed to check your retention of information related to the changes that occur in each stage of life. Feel free to read over the content notes in your learner resource related to this topic before attempting these questions.
H5P here
When a muscle imbalance is present, it is likely there because:
- A muscle or muscle group has become overactive or tight.
- A muscle or muscle group has become under-active or weak.
If left untreated muscle imbalances can lead to a number of negative outcomes including:
- Altered movement patterns and poor biomechanics
- Reduced range of motion
- Joint dysfunction & misalignment
- Increased risk of pain and injury – including overuse injuries
- Reduced athletic performance
Upper Crossed Syndrome
The term upper crossed syndrome encompasses a series of muscle imbalances that combine to form altered posture in the superior aspects of the torso, along with the head and neck. People presenting with this issue have rounder shoulders, an exaggerated curvature of the thoracic spine and a forward neck and head position.
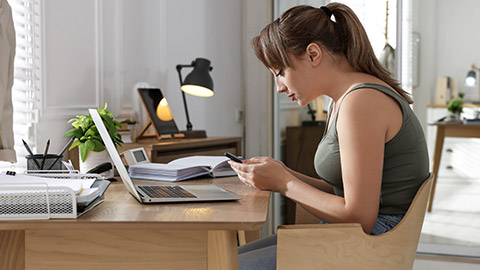
Upper crossed syndrome is becoming increasingly common in society due to the increased time spent sitting on electronic devices including computers and mobile phones. Holding these positions for extended periods of time leads to adaptive shortening of some muscles, that can alter postural causing reciprocal inhibition of functional antagonists (leading to them becoming weak or poorly recruited).
This series of questions is designed to assess your understanding of the muscle imbalances involved in upper crossed syndrome and the process for correcting them.
H5P here
Summary
While we will continue to explore common muscle imbalances and correction techniques for the remainder of the week in class using a second case study topic (Lower Crossed Syndrome), the learning in this topic you need to cover related to assessment 1 (part B) is now complete. There is one more topic to cover before we can complete this assessment. None of the organ systems we have covered so far can stay alive without a constant supply of oxygen and fuel. These key life-giving elements are delivered via a network of blood vessels which form the basis of our next topic: The cardiovascular system.
The remainder of your online content for this part of your online learning will focus on the key structures and functions of the cardiovascular system.
H5P here
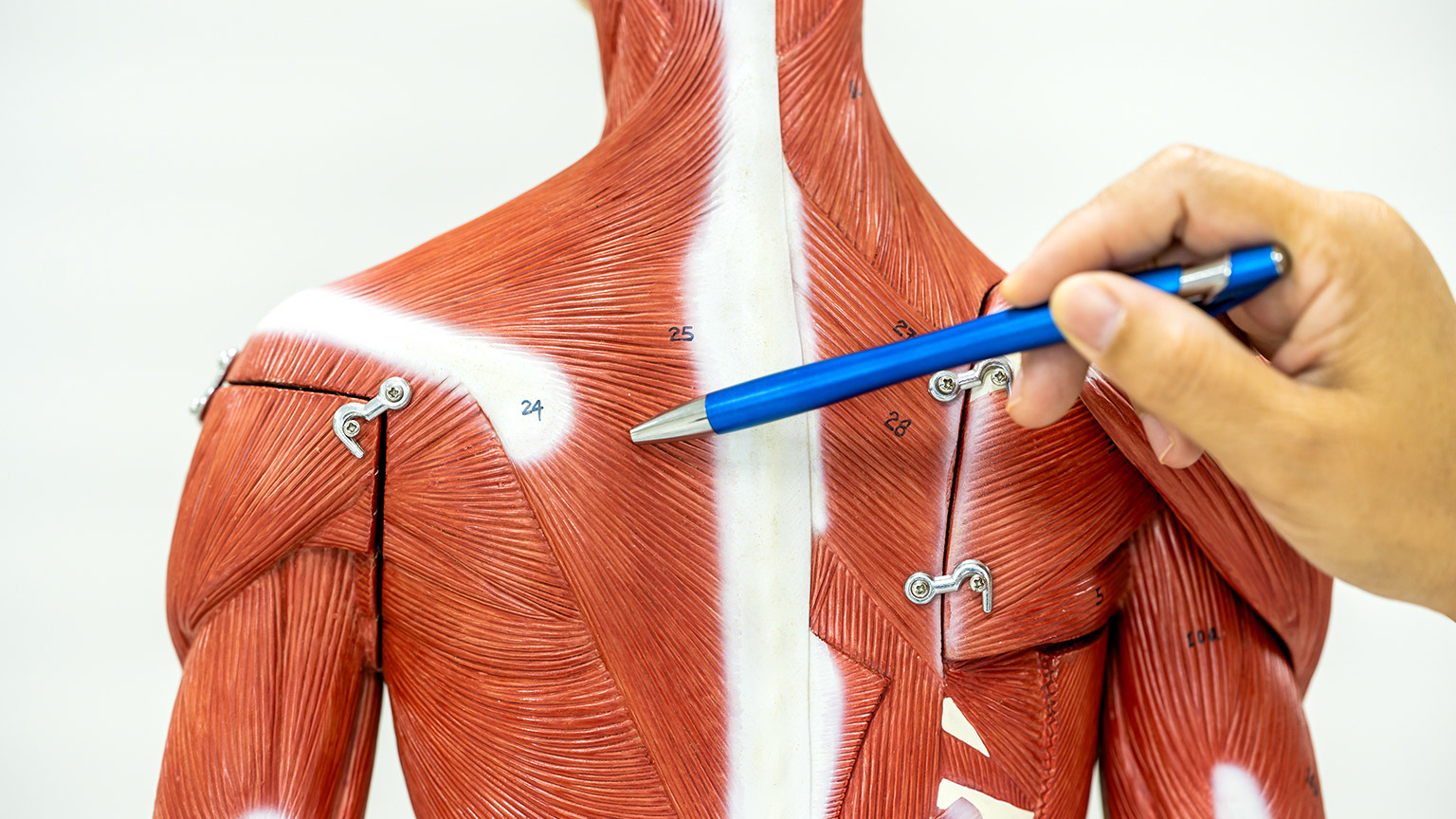