In this topic, we focus on the structure and function of the cardiovascular system.
The cardiovascular (CV) system is essential to every aspect of human life and athletic performance. The cardiovascular system (cardio~= heart, vascular~= blood or blood vessels) is composed of 3 components:
- The heart
- Blood vessels
- Blood
The role of the CV system is to provide essential nutrients to the body's cells and tissues when required and remove waste products from the body. We will look at the heart, blood vessels, and blood, and how they each contribute to athletic performance as well as the maintenance of homeostasis.
In this topic, you will learn about:
- The heart
- Blood vessels
- Blood
- Circulatory system
- Measurements of heart function
Watch
This short video shows the internal structure of the heart. Watch it to get familiar with the heart's structure before progressing.
The heart is the 'battery' of the cardiovascular system. Without the heart beating and pumping blood through our vessels, the CV systems would be unable to remove waste and deliver nutrients throughout the body.
Heart function
The heart has one primary function. For the body to stay alive, each cell must receive a continuous supply of nutrients and oxygen. At the same time, carbon dioxide and other materials produced by the cells must be transported away from the tissues and removed from the body.
Move blood heart and lungs
Move blood heart and body tissues
Pump blood around the body
Maintain homeostasis
These processes are continually maintained by the body’s cardiovascular system (circulatory system). It is the heart's job to produce the pressure required to move blood between the heart and lungs, and the heart and body tissues, pump blood around the body and maintain homeostasis.
Did you know?: Your heart beats approximately 10,000 times a day. This amounts to approximately 35 million beats in a year and 2.5 billion times in an average lifetime.
Heart structure
Your heart is roughly the same size (but not the same shape) as your fist and weighs approximately 250g for females and 300g for males.
Chambers
Our heart is composed of 4 separate chambers that contribute to how it performs its pumping action. There are 2 superior receiving chambers (the atria) and 2 inferior (below the atria) pumping chambers (the ventricles), with one of each on the right and left portions of the heart.
Right and left atria: Receiving chambers |
The right and left atria receive blood returning to the heart. The right atrium receives deoxygenated blood returning from the body tissues, while the left atrium receives oxygenated blood from the lungs. Between the right and left atria is a layer of tissue called the atrial septum that separates the left and the right receiving chambers. |
---|---|
Right and left ventricles: Pumping chambers |
The right and left ventricles are the heart pumps. They receive the blood from the corresponding atria via 'gateways' called valves. These valves open when there is sufficient pressure pushing against them, controlling the rate and flow of blood from the atria to the ventricles. The ventricles pump the blood to relevant parts of the body depending on the oxygen status of the blood. The right ventricle pumps deoxygenated blood to the lungs, while the left ventricle pumps oxygenated blood to the body tissues. |
Valves
4 valves separate the different chambers of the heart. They are controlled by the nervous system and work together to keep blood moving through the heart and around the body. Most importantly, they keep blood moving in the same direction.
The valves perform their function during the phases of a heartbeat.
- Systole - a contraction of the ventricles
- Diastole - relaxation of the ventricles
Coronary arteries
The heart is a specialised muscle, and like any muscle, the heart requires oxygen to stay alive. Even though our heart contains oxygen-rich blood, it cannot gain oxygen from the blood inside it. Instead, coronary arteries deliver O2 rich blood. These arteries branch off into smaller arteries that envelope the heart.
Typically, there is one artery on the right side and one on the left, with the left one generally being the larger. This is because the left side of the heart pumps blood to the body, whereas the right-hand side pumps blood to the lungs.
The left main coronary artery divides into 2 sizable branches, the left anterior descending (supplying the front of the heart) and the left circumflex (wrapping around the left side and back of the heart). The right coronary artery supplies the back of the heart.
Blockages of these vessels are one of the leading causes of death.
Muscular tissue of the heart: Myocardium
The heart is composed primarily of muscular tissue, specifically the myocardium.
When you look at a cross-section of the heart, you can see a subtle difference in the myocardium. The left-hand side of the heart (on the right), particularly around the ventricle, is substantially thicker than around the right ventricle. This is because the left ventricle pumps blood around the entire body, so it needs to be strong enough to create sufficient pressure to do so.
Heart muscle anatomy
How the heart beats
The cardiac muscles of the heart are referred to as autorhythmic fibres because they are self-excitable. This refers to the fact that they repeatedly generate their own action potentials that trigger contractions of the heart muscle fibres.
Heart conduction system
The heart conduction system is the network of nodes, cells, and signals that controls your heartbeat. Conduction follows a complex sequence that coordinates the pumping of each heart chamber at the right time. This allows blood to flow in the correct direction and with the appropriate amount of force, for example, the left ventricle must pump harder than the right to deliver blood to the body. This is controlled by your autonomic nervous system and is regulated by a system of chemoreceptors and electrical impulses.
Blood vessels are the pathways for blood around the body. There are 5 types of blood vessels:
- Arteries
- Arterioles
- Capillaries
- Venules
- Veins
Arteries
Arteries are the largest blood vessels and carry blood away from the heart. There are 2 types:
- Systemic: Carry blood from the aorta to be distributed to the body tissues. These carry only oxygenated blood.
- Pulmonary: Carry blood from the pulmonary trunk to be distributed to the alveoli in the lungs. These carry only deoxygenated blood.
Arteries branch at intervals along their length into arterioles which are tiny branches of arteries that lead to capillaries. Along with smaller arteries, these are under the control of the sympathetic nervous system and constrict (narrow) and dilate (widen) to regulate blood flow.
Arterioles
These are small structures that deliver blood to capillaries. Arterioles are the main effectors of blood pressure.
Capillaries
Capillaries are tiny, extremely narrow, blood vessels, approximately 5-20 micro-metres in diameter. They are so tiny, that red blood cells pass through in a single file line.
There are networks of capillaries in most of the organs and tissues of the body. Capillaries are supplied with blood by arterioles and are drained by venules. Capillary walls are very thin. This allows them to exchange substances and gasses between the blood and the surrounding tissue e.g., oxygen, carbon dioxide, water, and salts.
Venules
Venules are small vessels that drain blood from capillaries and into veins. Many venules unite to form a vein. They drain blood from capillaries and pass it on into veins for return to the heart.
Veins
Veins transport blood towards the heart. There are 2 types:
- Systemic: Carry deoxygenated blood back to the right atrium of the heart.
- Pulmonary: Carry oxygenated blood from the lungs back to the left atrium of the heart.
Veins have valves that prevent blood from flowing in the reverse direction.
Aside from both starting with the letter "b", how are bones and blood similar? Surprisingly, they are considered the same type of "connective tissue". Connective tissue is one of four types of tissue that make up the human body (the others include epithelium, muscle, and nervous) and has many functions. With that in mind, let us now discuss the components of blood.
Structure
Blood comprises 4 basic components: plasma (the liquid component) and 3 types of blood cells (the solid component).
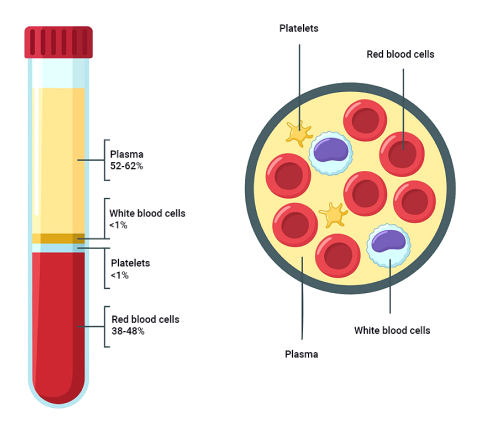
Plasma | A watery straw-coloured liquid containing ions, proteins, and hormones. It carries the blood cells around the body. |
---|---|
Red blood cells (erythrocytes) | These contain hemoglobin to transport oxygen. |
White blood cells (leukocytes) | Fights infection from illness and disease. Your body makes more white blood cells to fight bacteria and viruses when you are sick. This is why you might get a white cell count when unwell. |
Platelets (thromocytes) | Controls bleeding by clotting at wounds. |
The proportion of blood made up of cells is known as hematocrit. An average college-aged male has a hematocrit level of approximately 42%. An average college-aged female has a hematocrit level of approximately 38%. Hematocrit is largely determined by red blood cell numbers. If males have higher hematocrit, it follows that they also have a higher red blood cell count, so more oxygen can be delivered to muscles.
Watch
This short video describes the structure of plasma and the 3 blood cells, as well as what they do.
Function
As we've mentioned, blood transports essential goods like oxygen and nutrients to cells for their metabolic processes, and carbon dioxide and other wastes for excretion. But transportation is just one of four general functions.
Homeostasis of fluid balance
The cardiovascular system works with the lymphatic system to remove excess fluid from tissues, passing it to the urinary system for excretion in the urine. This plays a vital role in maintaining blood volume and maintaining the body’s fluid/water balance.
Thermoregulation (temperature control)
This term refers to the homeostatic regulation of the body’s temperature - the mechanisms our body uses to stay at its optimum temperature of 37.5 degrees Celsius. This is achieved by receptors in the body's skin detecting changes in heat.
Vasoconstriction | The vessels supplying blood to the skin will constrict (narrow). This decreases blood flow to the skin, minimising heat loss from warm blood. |
---|---|
Vasodilation | The vessels supplying blood to the skin will dilate (expand) to accommodate an increased volume of blood. This will cool the body. |
Have you ever noticed how skin changes colour when very hot or very cold? This is most noticeable when lips, fingers, or toes go blue in extreme cold, or when your face and body are flushed red in extreme heat or during exercise. For the following images, think about whether the blood vessels are constricted (vasoconstriction) or dilated (vasodilation).
Protection
When you get a cut, platelets bind to the site of the damaged vessel. This causes a blood clot. The plug-type function of platelets prevents severe blood loss from our bodies. Excess blood loss can result in conditions such as shock which can, in worst-case scenarios lead to full body shutdown and death. So, the clot-forming properties of blood are vital in preventing large volumes of blood loss.
White blood cells are part of the body's immune system. They help the body fight infection and other diseases by identifying and attacking unknown organisms and carrying them away in the blood for removal.
Transportation
Blood is the sole distributor of oxygen in our bodies. Oxygen binds to hemoglobin in red blood cells and is transported from the lungs to the tissues in the body. Red blood cells also pick up carbon dioxide (a waste product) from the tissues. This is pumped back to the lungs via the heart for exhalation and removal from the body.
Blood carries nutrients (from our gastrointestinal tract) and hormones (from the glands) to the body cells. Blood also carries wastes to the kidney and liver for detoxification and removal. Finally, blood carries heat to the skin.
Blood follows a certain pattern through vessels in and out of the heart.
Deoxygenated and oxygenated blood
In anatomical diagrams, you will usually see blood depicted in two colours: blue and red.
- Blue represents deoxygenated blood. This blood lacks oxygen O2. It is heading back to the lungs to receive oxygen inhaled from the environment.
- Red indicates oxygen-rich (oxygenated) blood. This blood has been oxygenated in the lungs and is now on its way to the tissues and cells of the body.
All the body's cells need oxygen to efficiently create energy and perform tasks such as digestion, muscle contraction, and cell communication.
Take a look at the following image, which highlights the direction of travel of blood both into and out of the heart.
Remember that when you look at a picture of a heart front-on, the left is on the right, and the right is on the left.
The pathway of blood through the heart is of great physiological importance when working in industries such as health and fitness. Becoming comfortable with the direction of blood, and the factors involved in pumping blood through the heart and the body, will help you design the best programmes for your clients. Understanding the circulatory system and having the ability to calculate capacities suitable for your clients, are incredibly valuable tools to draw on when you design a tailored routine for your clients.
Watch
This video goes through the steps of how blood flows through the heart. It's only a couple of minutes long, but you may want to watch it a few times to get really comfortable with it.
Let's look at the pathway of blood through the heart.
Deoxygenated blood flow
This is known as the pulmonary circuit and happens on the right side of the heart.
- Deoxygenated (oxygen-poor) blood arrives in the right atrium (receiving chamber) from the superior vena cava (upper body) and the inferior vena cava (lower body).
- Blood gathers in the right atrium, which applies pressure to the atrium walls and the tricuspid valve.
- When a sufficient volume of blood arrives in the right atrium, the tricuspid valve opens, and blood then enters the right ventricle.
- When the ventricle is full, pressure is applied to the rear of the tricuspid valve, which closes the tricuspid valve. This prevents the backflow of blood while the ventricle contracts.
- The right ventricle contracts, pushing blood out of the ventricle, and up the pulmonary artery. This is enabled by the opening of the pulmonary valve.
- This deoxygenated blood then arrives, via the pulmonic artery to the lungs. Gas exchange takes place and the blood is refreshed with oxygen, and carbon dioxide is removed through exhalation.
At the same time as deoxygenated blood is flowing through the heart, oxygenated blood is also flowing through.
Oxygenated blood flow
This is known as the systemic circuit and happens on the left side of the heart.
- Oxygen-rich blood arrives via the pulmonary vein and empties into the left atrium.
- When sufficient blood is gathered here, producing sufficient pressure on the mitral valve, the mitral valve opens.
- Blood flows from the left atrium into the left ventricle via the mitral valve.
- When the left ventricle is full of blood, the pressure of this blood pushes back on the mitral valve. This forces it to close and protects against backward blood flow into the atria while the ventricle contracts.
- As the ventricle contracts, blood is propelled into the aorta via the aortic valve. It then travels into the veins and onwards to the body's cells.
Watch
Now is a great time to watch this video, it nicely sums up what we have discussed. Exhale, take a break, and enjoy the next few minutes.
There are many methods available to assess heart function. Some require invasive technology or instruments which enter the body. In the health and fitness industry, you will use non-invasive techniques to measure heart function. In this topic, we will go through some typical calculations you may do as a personal trainer. Practice using Excel or a calculator to complete the calculations.
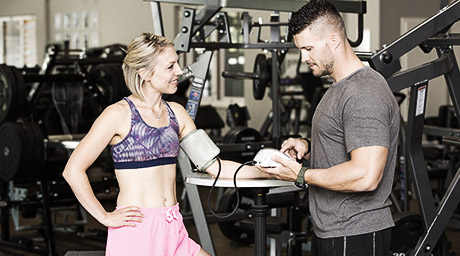
Blood pressure
Blood pressure (BP) is the force exerted by circulating blood on the walls of blood vessels during the heart contraction cycle (both contraction and resting phases).
A minimum blood pressure is required to ensure adequate blood flow to all parts of the body, especially the brain. The term blood pressure generally refers to arterial pressure, i.e., the pressure in the larger arteries.
Blood pressure is measured in 2 parts.
- Systolic: the maximum pressure reached in the arteries during the ventricular contraction phase of the heartbeat.
- Diastolic: the minimum pressure recorded just before ventricular contraction (resting phases).
Blood pressure is generally recorded as systolic/diastolic (this means systolic over diastolic).
To measure blood pressure, a cuff is placed around the client or patient's arm and inflated. The measurement can be taken manually using a stethoscope or using a device that measures pressure. Blood pressure is measured in mmHg (millimetres of mercury).
Normal systolic pressure is between 110-130 mmHg and normal diastolic pressure is between 70-80 mmHg (Health Navigator NZ, 2022). The New Zealand Heart Foundation say 120/75 mmHg is the ideal blood pressure for most people. If a client has very high blood pressure, do not train until cleared by a physician (GP).
Systolic | Diastolic |
---|---|
Less than 120 A healthy result, recheck in a year |
Less than 80 |
Between 120-129 Monitor as there is a high chance of your blood pressure increasing |
Less than 80 |
Between 130-139 A high result, usually treated with lifestyle changes. Medication may be suggested if you have other stroke risk factors |
Between 80-90 |
Greater than 140 Usually treated with medication and lifestyle changes |
Greater than 90 |
Greater than 180 Needs urgent attention right away |
Greater than 120 |
Blood Pressure Table by Stroke Foundation NZ, © Stroke Foundation NZ
Watch
The following video demonstrates how to take a manual blood pressure reading. You may need to watch it a few times to get familiar with the steps.
It's also possible to use a device that gives you an automatic reading. Watch the following video for a demonstration using an automated blood pressure cuff.
Peripheral vascular resistance
Peripheral vascular resistance (also known as systemic vascular resistance, SVR) is the resistance in the circulatory system that is used to create blood pressure, the flow of blood, and is a component of cardiac function. Vasoconstriction results in an increase in SVR. SVR depends on:
- the length of the vessel: Longer vessels take more pressure to push blood through.
- the radius of the vessel: A small change in vessel diameter can have a dramatic impact on resistance! E.g., if your vessels become narrower (this may be due to vasoconstriction or cholesterol), your BP will rise.
- the viscosity of the blood: The thicker the blood, the harder the heart has to work to move it, so BP rises.
Effects of exercise on blood pressure
One of the acute effects of exercise on the body is an increase in systolic blood pressure. Systolic blood pressure will increase during exercise and stabilise 2 - 3 minutes after the start of exercise. Diastolic blood pressure should remain relatively unchanged as it represents the resting phase of the heart cycle.
A chronic effect of endurance exercise is a gradual lowering in BP due to a reduction in sympathetic nervous system activity and reduced peripheral resistance.
The acute effect of strength training on BP is a sharp rise in systolic BP while lifting. This can often be made worse due to the Valsalva maneuver (this is a long version of what you might do on a plane to pop your ears). During intense lifting, the glottis may close as you hold your breath. This increases pressure in abdominal and thoracic cavities inhibiting blood return. It can be very dangerous for those with cardiovascular disease, high blood pressure, or the elderly.
Hearts change after chronic aerobic training. Chronic aerobic training results in an increase in heart strength and size due to cardiac hypertrophy. The increase in heart strength allows for an increase in stroke volume (blood flow/pumped per heart contraction). Therefore, the overall heart rate is lower as the heart is not having to beat as fast to circulate the same amount of blood through the circulatory system. Overall cardiac output (stroke volume x heart rate) increases which benefits performance as more oxygenated blood can be provided to the working muscles at a decreased workload. This allows for more efficient exercise and greater workloads able to be achieved.
If a client has high resting blood pressure, it will increase significantly while exercising. This can be very dangerous. The client could pass out or suffer cardiac arrest. It is important that you measure every client's blood pressure and refer them to a doctor if it is high. We'll look at pre-screening and referral later in the course, but it is worth noting the importance of this now.
Cardiac output (Q)
Cardiac output is the amount of blood ejected from the left ventricle to the body in one minute. It is worked out using the following equation:
Q = HR x SV
Where:
- Q is cardiac output in millilitres per minute (mL/min), often converted to litres per minute (L/min)
- HR is heart rate in beats per min (beats/min or BPM)
- SV is stroke volume in millilitres per beat (mL/beat) - this is a measure of the amount of blood ejected in each beat
At rest, cardiac output is about 5L/min but during max exercise, it can exceed 35L/min!
Let's work through an example.
In a typical adult resting male, heart rate (HR) is approximately 75 beats/min and stroke volume (SV) averages 70mL/beat. Using the equation Q = HR x SV we can calculate the cardiac output:
Q = HR x SV
Q = 75 BPM x 70mL/beat
Q = 5,250 mL/min
This can be converted to L/min by dividing by 1,000. This gives us Q = 5.25 L/min.
Blood distribution at rest vs during exercise
Beginning exercise, or increasing exercise intensity, usually requires rapid adjustments in blood flow that affect the entire cardiovascular system. The main differences in blood distribution when transitioning from rest to exercise:
- Increased blood flow to working skeletal muscles and skin
- Reduced blood flow to less active organs (e.g., digestive system)
- At rest, 15% - 20% of total Q (cardiac output) goes to muscles (postural)
- During exercise, 80% - 85% of total Q goes to muscles
This is illustrated in the following charts:
- increased HR: This is typically a linear increase to match the increased demands of tissues for blood flow.
- increased SV: This rises initially due to heart stretch as more blood is returned to the heart from working muscles. It plateaus at about 40% VO2max (maximal amount of oxygen consumption). After this, all increases are due to a rise in HR.
Stroke volume (SV)
This is the amount of blood pumped from each ventricle in one beat in millilitres. It is governed mainly by 3 factors:
- Preload: The amount of stretch on the heart, before it contracts (think of a stretched elastic band before you let it go).
- Contractility: The strength/forcefulness of the contraction.
- Afterload: The blood pressure outside the arteries vs inside the ventricles and heart vessels. Blood flows from an area of high blood pressure to an area of low blood pressure. It is the balance of these pressures assisting in the speed of blood flow.
During exercise stroke volume increases due to an increase in venous return. Venous return is blood flow returning to the heart from working muscles. This leads to a more complete filling of the heart followed by a more forceful ejection and therefore, greater stroke volume.
As a personal trainer, you cannot measure stroke volume. However, you can understand that an average stroke volume is around 70mL per beat (this could change depending on your client's size and other factors). The point is that as your heart gets stronger and more efficient, your stroke volume will increase over time. Your heart will be better at pumping blood around the body delivering the nutrients it needs (i.e., oxygen and glucose) so you can perform better.
Heart rate (HR)
During exercise heart rate increases due to the increased demand for blood flow by working muscles. Muscles require more oxygen and nutrient delivery and more waste removal (heat, CO2). A normal resting heart rate is between 60 and 100 BPM.
Newborn infants have higher heart rates (often 120 BPM or greater). This is due to smaller stroke volume (and less total blood).
Watch
The following video shows how to check your heart rate (pulse). Watch the video, then take your own pulse.
If you cannot find your pulse using the radial artery in your wrist, you can try the carotid artery in your neck.
Maximum HR (HRmax or MHR)
This is simply defined as the maximum number of beats your heart can pump per minute under maximum stress. There are several different methods to calculate MHR. We'll look at the most popular.
HRmax = 220 - age (years)
For example, if you were 36 years old, the calculation would be:
HRmax = 220 - age
HRmax = 220 - 36
HRmax = 184 BPM
A good grasp of these calculations enables you to programme accurately and safely.
Heart rate and exercise
HR increases linearly with exercise intensity up to a maximum. Once VO2max (maximal amount of oxygen consumption) has been reached, HR levels off.
During prolonged exercise, there will be an initial rise in HR, which will reach a steady state after 2 to 3 minutes, if the intensity is constant and at a sub-maximal level.
A chronic effect of exercise is a decrease in HR at any given exercise intensity.
Cardiac output and exercise
The following table shows that while untrained and trained males of the same size require the same amount of blood (Q = 5 L/min), they get it differently. Note that the trained male achieves his 5L/min with a much lower resting heart rate. This is due to having a much stronger heart, capable of ejecting more blood per beat with higher SV. The same is also true of females.
Subject | HR (BPM) | SV (mL/min) | Q (L/min) |
---|---|---|---|
*REST | |||
Untrained male | 72 | 70 | 5.00 |
Untrained female | 75 | 60 | 4.50 |
Trained male | 50 | 100 | 5.00 |
Trained female | 55 | 80 | 4.50 |
The following table shows that a major advantage of being fit is when working at high intensities you can deliver far more blood to working muscles (34 L/min compared to 22 L/min in fit vs unfit males). This is a huge advantage allowing you to deliver more oxygen and fuel to working muscles, and crucially, to be able to remove waste more effectively, so you can maintain higher intensities for longer. The same is true in females but to a lesser extent. This is mainly due to them having a slightly smaller heart-to-body size ratio.
Subject | HR (bpm) | SV (ml) | Q (L/min) |
---|---|---|---|
*MAX EXERCISE | |||
Untrained male | 200 | 110 | 22.0 |
Untrained female | 200 | 90 | 18.0 |
Trained male | 190 | 180 | 34.2 |
Trained female | 190 | 125 | 23.9 |
During prolonged exercise:
- Q stays the same
- HR increases
- SV decreases
SV decreases due to loss of sweat causing a dehydration effect as blood plasma is lost in sweat (unless rehydration is adequate). This means less blood is returned to the heart causing a lower SV. HR must climb to compensate.
This is even more pronounced in warm conditions and is known as 'cardiovascular drift'.
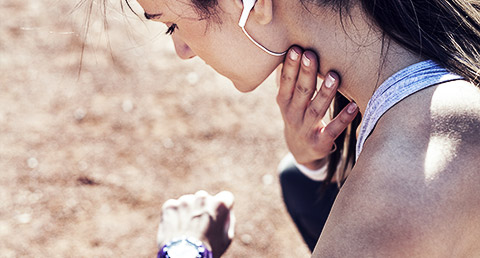
Heart Rate Reserve (HRR) and training zones: The Karvonen Formula
The Karvonen method, also known as the heart rate reserve (HRR) formula, takes your resting heart rate into consideration and analyses the difference between this and your maximum heart rate to calculate what is known as your 'Heart Rate Reserve' in accordance to calculating individualised target heart rate parameters, e.g., 60% max intensity.
In short, the heart rate reserve is the sum of the difference between your maximum heart rate and your resting heart rate. To produce a more accurate calculation, your resting heart rate should be calculated as the average sum of 3 consecutive readings, (ideally 3 mornings in a row directly after getting out of bed). This is not always possible in a gym setting, so to avoid sending your clients home three days in a row, simply measure three consecutive readings.
The Karvonen Formula helps you determine a target heart rate training zone.
Target taining HR = resting HR + (intensity x [maximum HR - resting HR])
Where:
- Resting heart rate (RHR) = pulse at rest
- Maximum heart rate (MHR) = 220 - age
- Heart rate reserve (HRR) = MHR - RHR
- Intensity is training percentage as a percentage (this can be shown as a decimal, e.g., 60% is 0.6)
Let's work through an example together. A 36-year-old female with a resting heart rate of 56 bpm wants to work out at 60% of her maximum capacity.
Target training HR = 56 + [(220-36) − 56) × 0.6]
Target training HR = 56 + [184 − 56) × 0.6]
Target training HR = 56 + [128 × 0.6]
Target training HR = 56 + 76.8
Target training HR = 132.8 bpm (rounded to 133 bpm)
Note: If the above calculation seems tricky due to the order of operations, you may like to review BEDMAS.
Recovery zone: 60 - 70% | Active recovery training should fall into this zone (ideally to the lower end). It's also useful for very early pre-season and closed-season cross-training when the body needs to recover and replenish. |
---|---|
Aerobic zone: 70 - 80% | Exercising in this zone will help to develop the aerobic system and in particular the ability to transport and utilise oxygen. Continuous or long, slow-distance endurance training should fall in this heart rate zone. |
Anaerobic zone: 80 - 90% | Training in this zone will help to improve the body's ability to deal with lactic acid. It may also help to increase the lactate threshold. |
Watch
Watch this brief yet informative video for an overview of the Karvonen method. This will be used frequently within your training programme design so it is important to become familiar with it.
Check that you have a good understanding of the cardiovascular system before moving to the next topic. You'll be assessed on the cardiovascular system in a combined test with the respiratory system.
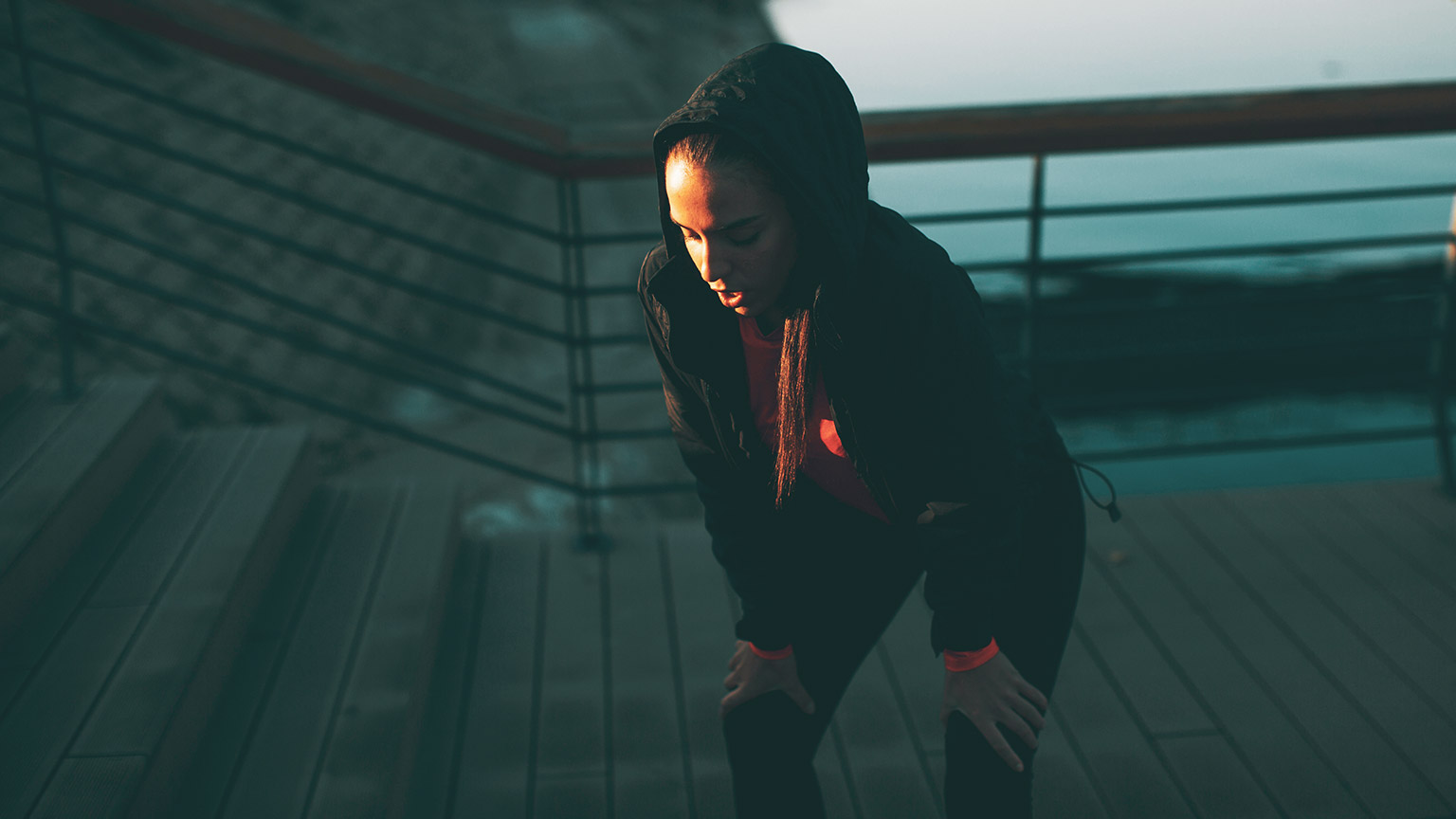